In a crystal, collective excitations can be treated as if they are quasiparticles. For example, an excited electron and its associated hole are an exciton; oscillations of conduction electrons in a metal are plasmons; and different modes of lattice vibrations are phonons. Furthermore, when such excitations interact strongly with photons, a new form of quasiparticle emerges: the “polariton.” The ability to excite and control polaritons in two-dimensional materials is enticing because it offers the prospect of sidestepping the diffraction limit and manipulating light at subwavelength scales for nanophotonics applications. At the ALS, researchers have used synchrotron infrared nanospectroscopy (SINS) to study the behavior of phonon polaritons in ultrathin crystals of hexagonal boron nitride (hBN). The results pave the way towards engineering infrared-light photonic nanodevices and expand our understanding of polariton behavior in low-dimensional nanomaterials.
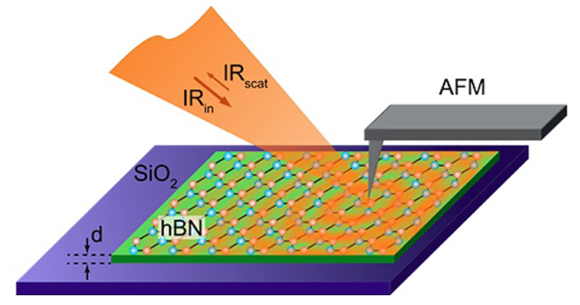
SINS is a revolutionary infrared (IR) technique developed at ALS Beamline 5.4.1 by a collaboration between the University of Colorado at Boulder and the ALS. In this technique, IR light is focused onto the metal tip of an atomic force microscope. As it scans over the sample, the tip acts as an antenna, directing the light onto a tiny region of the sample (the near field), generating polaritons. Polariton waves propagate outward until reflected from the sample edge. The back-reflected waves interfere with the excitation field at the tip, modifying the amplitude and the phase of the tip-scattered near field, which is then detected for spatial and spectral imaging. With a spatial resolution up to a thousand times better than conventional Fourier-transform infrared (FTIR) spectromicroscopy, SINS enables the investigation of nanoscale phenomena in soft matter, even under ambient and environmental conditions that are essentially inaccessible by other techniques.
In earlier experiments at the ALS using a laser as a source, the researchers were able to study other low-dimensional materials such as bilayer graphene and carbon nanotubes. In the graphene, they discovered topologically protected one-dimensional electron-conducting channels at domain walls that were “valley polarized,” a feature that could prove useful in future quantum-computing devices. In the carbon nanotubes, they were able to directly observe “Luttinger liquid” plasmons for the first time, with wavelengths only one hundredth the size of the free-space photon wavelength, and one of the strongest field-confinement factors ever reported.
In this work on hBN, the researchers used the ALS itself as a source of broadband IR light. The large spectral bandwidth of the synchrotron beam enabled the measurement of both out-of-plane (780 cm−1) and in-plane (1370 cm−1) hBN phonon modes and the measurement of both phase and amplitude simultaneously. Synchrotron IR radiation is currently the only source with the combined spectral irradiance, spectral coverage, and high repetition rate to measure near-field spectra over the entire mid-IR range within a single acquisition. Other advantages include minimal shot-to-shot fluctuations, high long-term power stability, and exceptionally stable spectral distribution. These advantages enable effective signal averaging over minutes to hours, if necessary, to improve the signal-to-noise ratio because the near-field signal is much weaker relative to the back-scattered far-field background.
Several hBN specimens with thicknesses varying from 3 to 160 nm were measured and analyzed. The results showed that the in-plane phonon polariton is much stronger than the out-of-plane phonon polariton. More interestingly, the polariton wavelength was found to be proportional to the sample thickness. The researchers developed a simple theory to describe this proportional dependence. This simple model correctly predicts the linear scaling behavior between phonon polariton wavelength and sample thickness for a given frequency in thin hBN crystals. The researchers expect this simple model to provide a good general description of any two-dimensional polariton system, including plasmon polaritons in graphene, as well as phonon or exciton polaritons in other ultrathin crystals.
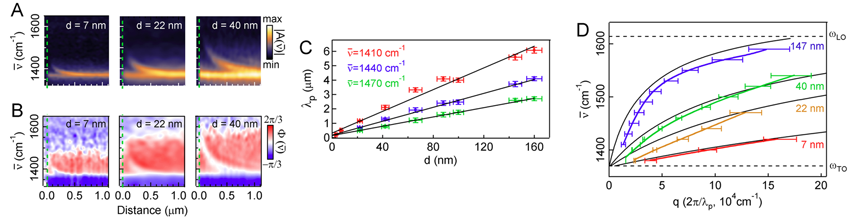
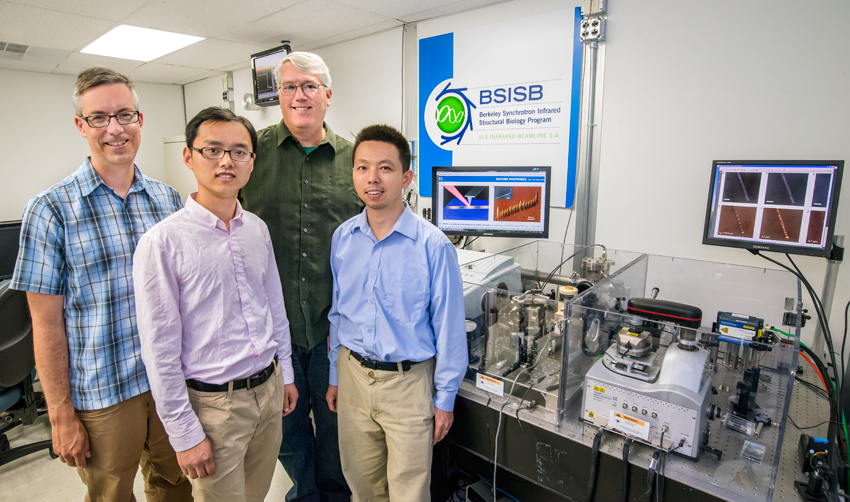
Contact: Feng Wang
Research conducted by: Z. Shi, Y. Sun, B. Zeng, C. Jin, and H. Chang (University of California, Berkeley); H.A. Bechtel and M.C. Martin (ALS); S. Berweger (NIST and University of Colorado, Boulder); M.B. Raschke (University of Colorado, Boulder); and F. Wang (UC Berkeley and Berkeley Lab).
Research funding: Office of Naval Research; David and Lucille Packard Foundation; and U.S. Department of Energy (DOE), Basic Energy Sciences (BES) and Biological and Environmental Research (BER) Programs. Operation of the ALS is supported by DOE BES.
Publications about this research: Z. Shi, H.A. Bechtel, S. Berweger, Y. Sun, B. Zeng, C. Jin, H. Chang, M.C. Martin, M.B. Raschke, and F. Wang, “Amplitude- and Phase-Resolved Nanospectral Imaging of Phonon Polaritons in Hexagonal Boron Nitride,” ACS Photonics 2, 790 (2015); L. Ju, Z. Shi, N. Nair, Y. Lv, C. Jin, J. Velasco Jr, C. Ojeda-Aristizabal, H.A. Bechtel, M.C. Martin, A. Zettl, J. Analytis, and F. Wang “Topological valley transport at bilayer graphene domain walls,” Nature 520, 650 (2015); and Z. Shi, X. Hong, H.A. Bechtel, B. Zeng, M.C. Martin, K. Watanabe, T. Taniguchi, Y.-R. Shen, and F. Wang, “Observation of a Luttinger-liquid plasmon in metallic single-walled carbon nanotubes,” Nature Photonics 9, 515 (2015).
ALS SCIENCE HIGHLIGHT #325