Using synchrotron infrared nanospectroscopy (SINS) at the ALS, researchers have for the first time probed infrared plasmons (oscillations of free electrons) in single nanocrystals of doped metal oxides. Because each nanocrystal is much smaller than an infrared wavelength, plasmons in such nanocrystals could previously only be probed collectively rather than individually, averaging out details of interest. With the single-nanocrystal resolution offered by SINS, the researchers found that the nanocrystals generally perform better than previously assumed and that rational dopant selection, guided by SINS data, will allow the production of more-efficient plasmonic devices for molecular-sensing and energy-harvesting applications.
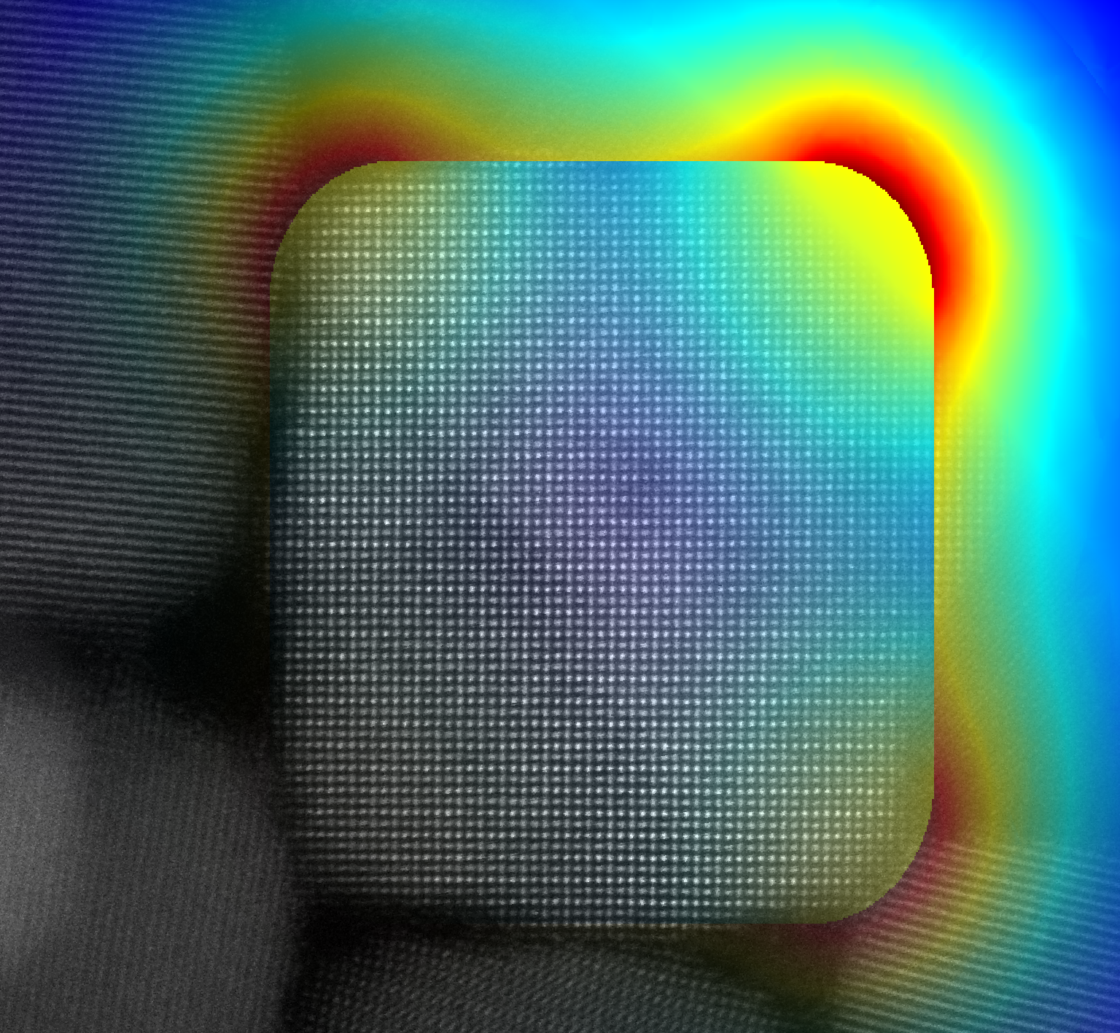
In metal oxide nanocrystals, n-type doping generates free electrons that can particpate in infrared-driven surface oscillations. These localized surface plasmon resonances (LSPRs) concentrate far-field radiation into near-field “hot spots” at energies where biological environments are transparent, molecular vibrations are resonant, and solar energy is abundant. Thus, these materials are attractive for a wide range of applications, from molecular sensing to high-efficiency organic solar cells. The development of such applications hinges on maximizing the near-field enhancement. One way to achieve this is by minimizing the damping of the plasmon oscillation.
At the University of Texas, Delia Milliron and her group have been working to expand the library of plasmonic metal oxide nanocrystals and to understand the ways in which their behavior differs from more traditional metal plasmonic systems. At the ALS, the group performed SINS studies of two well-known metal oxide materials, aluminum-doped zinc oxide (AZO) and tin-doped indium oxide (ITO), as well as a novel material synthesized in their lab, cerium-doped indium oxide (ICO).
The SINS instrument developed at the ALS (available at Beamline 5.4) allows the collection of mid-infrared spectra with a spatial resolution (~20 nm) capable of isolating single nanocrystals. Unlike similar laser-based experiments, the ALS offers bright, broadband infrared light, which allows for the unambiguous collection of complete LSPR spectra in a single measurement without the systematic error that would be introduced by tuning a laser source several times to collect a spectrum from a nanocrystal with a broad spectral feature. Furthermore, the linewidth of an LSPR peak from a homogeneous sample provides a convenient quality factor because it is proportional to plasmon damping: narrower peaks correspond to weaker damping, longer plasmon lifetimes, and stronger near-field intensities.
In the AZO and ITO experiments, the researchers found that single-nanocrystal spectra had linewidths less than half that of ensemble-averaged samples, indicating significant particle-to-particle variation in dopant integration. Although the researchers expected that particle heterogeneity, as opposed to plasmon damping, was leading to substantial broadening of the ensemble LSPR linewidth, they were surprised to find that metal oxide nanocrystals actually exhibit substantially lower damping than metal nanocrystals, allowing them to be extremely high-performing in the mid-infrared region.
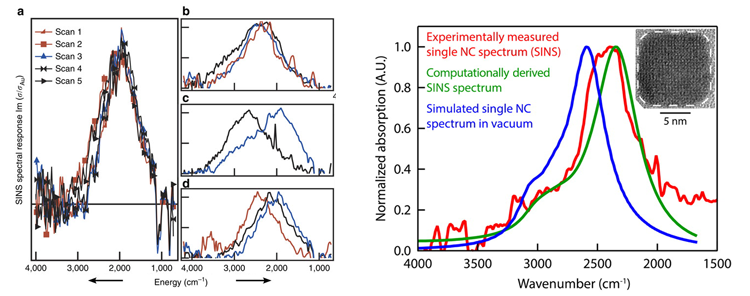
In a later experiment, the researchers synthesized ICO nanocrystals, adapting a low-cost, solution-processable procedure used for ITO but replacing the tin dopant with cerium, a more energetically stable electron donor and a better fit in the crystal lattice. The nanocrystals adopted cubic or pseudospherical shapes depending on the amount and type of cerium precursor used. From the SINS spectrum of a single cubic ICO nanocrystal, the researchers were able to simulate its absorption, scattering, and near-field properties. The results showed that the near-field enhancement was strongest at the cube vertices and was much stronger overall compared to the pseudospherical nanocrystals. With the SINS-enabled ability to nanofocus infrared light down to the nanoscale, the rational dopant selection strategies exemplified in this work could in the future be applied to any number of doped metal oxides.
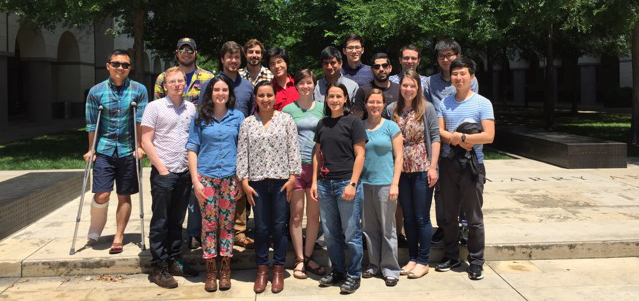
Contact: Robert W. Johns
Research conducted by: R.W. Johns and E.L. Runnerstrom (UC Berkeley and UT Austin); H.A. Bechtel (ALS); S.D. Lounis (UC Berkeley); A. Bergerud (Norwegion Univ. of Science and Technology, UC Berkeley, and UT Austin); S.M. Selbach (Norwegian Univ. of Science and Technology); and A. Agrawal, C.J. Dahlman, A. Singh, and D.J. Milliron (UT Austin).
Research funding: US Department of Energy (DOE) Small Business Innovation Research (SBIR) Program, DOE Early Career Research Program, DOE ARPA-E, Welch Foundation, Norwegian High-Performance Computing Consortium (NOTUR), National Science Foundation, DOE Office of Energy Efficiency and Renewable Energy, UC Berkeley Chancellor’s Fellowship, and ALS Doctoral Fellowship in Residence Program. Operation of the ALS is supported by the DOE Office of Basic Energy Sciences.
Publications about this research: R.W. Johns, H.A. Bechtel, E.L. Runnerstrom, A. Agrawal, S.D. Lounis, and D.J. Milliron, “Direct observation of narrow mid-infrared plasmon linewidths of single metal oxide nanocrystals,” Nat. Commun. 7, 11583 (2016); and E.L. Runnerstrom, A. Bergerud, A. Agrawal, R.W. Johns, C.J. Dahlman, A. Singh, S.M. Selbach, and D.J. Milliron, “Defect Engineering in Plasmonic Metal Oxide Nanocrystals,” Nano Lett. 16, 3390 (2016).
ALS SCIENCE HIGHLIGHT #337