SCIENTIFIC ACHIEVEMENT
The origin of the metal-to-insulator transition in a key material system was revealed by nanostructures designed to decouple simultaneous phase transitions.
SIGNIFICANCE AND IMPACT
This approach could lead to new materials with emergent physics and unique electronic properties, supporting broader research efforts to revolutionize modern electronics.
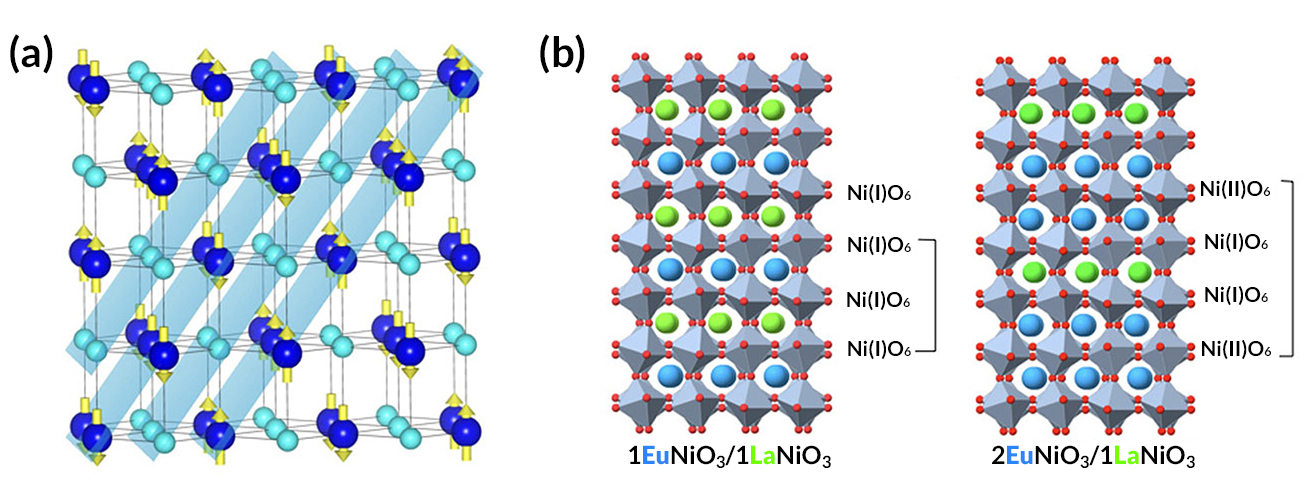
Emergent phenomena
When atoms or molecules assemble to form bulk matter, new properties (such as conductivity and ferromagnetism) that didn’t exist in the constituent parts can emerge from the whole. Similarly, stacking atomically thin layers into nanostructures (heterostructures) can give rise to a rich variety of emergent phases not found in bulk materials.
Materials that exhibit emergent phenomena (“quantum materials”) often feature multiple phases with simultaneous phase transitions. A great deal of effort is currently being expended to disentangle such transitions, to discover what drives them and to ultimately harness them in new materials with desired functionalities. Most of these efforts have relied on external perturbations (light, pressure, etc.) to decouple the transitions. In this work, researchers found a way to do this intrinsically, through layer-by-layer design of stacking sequences with mismatched periodicities.
An archetype of complexity
Rare-earth (RE) nickelates (RENiO3) are prototypical hosts of intertwined phase transitions. They include a metal-to-insulator transition (MIT) and transitions involving antiferromagnetic order and charge order. Charge ordering occurs when a pattern is formed (e.g. stripes or checkerboard) by nickel ions with different oxidation states (differences in the number of local electrons).
Earlier work on the origins of the MIT in such materials had given conflicting results. In this work, researchers attempted to clarify the picture by engineering materials to selectively supress the charge-ordering transition. They combined two different RENiO3 thin-film materials—EuNiO3 (ENO) and LaNiO3 (LNO)—in such a way that the periodicity of the designed structure did not match the periodicity required for stabilizing the charge ordering—a difficult technical challenge requiring subsequent laboratory and synchrotron-based characterization to demonstrate that the proper RE-site ordering was achieved with minimal intermixing.
Resonant soft x-ray diffraction at the ALS

The specific nature of the magnetic ordering and the corresponding transition temperature were revealed by resonant soft x-ray diffraction (RSXD) experiments at ALS Beamline 4.0.2. RSXD probes the periodic order of electronic and magnetic states in a material by diffracting x-rays into patterns characteristic of the electronic order.
The Beamline 4.0.2 scattering endstation is uniquely optimized for RSXD experiments on correlated oxides covering a wide range of scattering geometries and sample temperatures. Moreover, the beamline provides circular as well as variable linear polarization in the important transition-metal L3,2 edges as well as the oxygen K edge, allowing the team to disentangle multiple order parameters.
The ALS experiments showed that, in the heterostructure with mismatched periods, separation between the magnetic-ordering transition temperature and the metal-to-insulator transition temperature was achieved. In addition, experiments performed at the Advanced Photon Source revealed that the charge ordering was indeed suppressed in the mismatched sample. Taken together, the results emphasize that neither magnetic ordering nor charge ordering are necessary for the metal-to-insulator transition, pointing to one particular mechanism (known as the site-selective Mott transition) as being operative.
The researchers expect that this ability to effectively decouple simultaneous ordering phenomena in quantum materials with a simple stacking recipe will spur more discoveries on the nature of entangled orderings and could, in the long term, lead to unique functionalites and emergent physics not seen in bulk systems.
Contacts: Srimanta Middey and Derek Meyers
Researchers: S. Middey (Indian Institute of Science, India); D. Meyers (Brookhaven National Laboratory); M. Kareev, Y. Cao, X. Liu, and J. Chakhalian (Rutgers University); P. Shafer (ALS); and J.W. Freeland, J.-W. Kim, and P.J. Ryan (Argonne National Laboratory).
Funding: Gordon and Betty Moore Foundation; Indian Institute of Science; and U.S. Department of Energy, Office of Science, Basic Energy Sciences Program (DOE BES). Operation of the ALS is supported by DOE BES.
Publication: S. Middey, D. Meyers, M. Kareev, Y. Cao, X. Liu, P. Shafer, J.W. Freeland, J.-W. Kim, P.J. Ryan, and J. Chakhalian, “Disentangled Cooperative Orderings in Artificial Rare-Earth Nickelates,” Phys. Rev. Lett. 120, 156801 (2018), doi:10.1103/PhysRevLett.120.156801.
ALS SCIENCE HIGHLIGHT #379