SCIENTIFIC ACHIEVEMENT
Researchers using the Advanced Light Source (ALS) discovered how pure spin currents (also known as spin waves) can be efficiently and coherently transmitted through an electrically insulating antiferromagnetic material.
SIGNIFICANCE AND IMPACT
The work represents a notable milestone in the use of antiferromagnetic materials for low-power spintronic devices at room temperature.
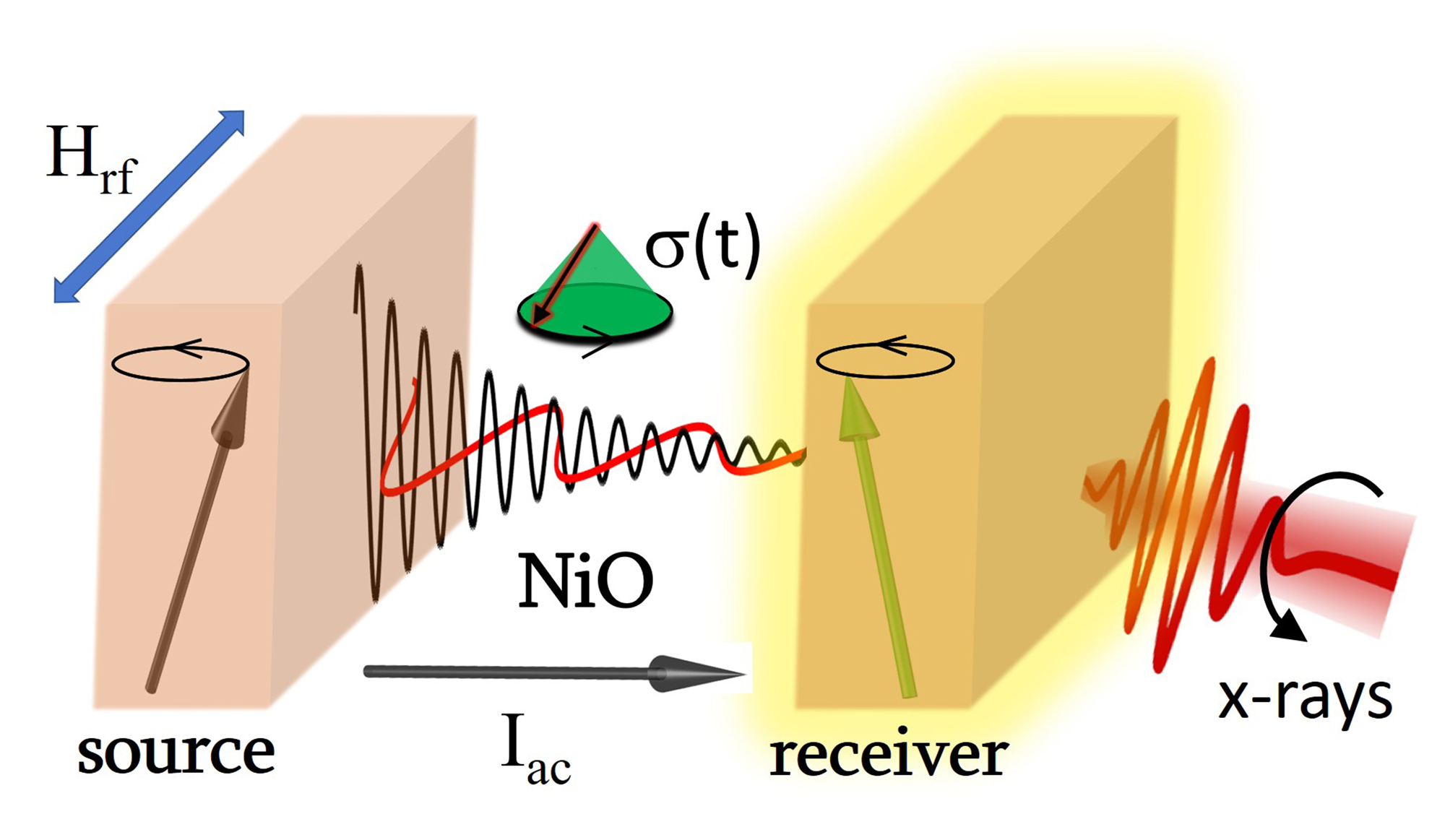
Electrically insulating, yet spin conducting
Antiferromagnetic (AFM) materials, thanks to their insensitivity to stray magnetic fields and ability to function at ultrafast terahertz frequencies, are highly promising candidates for future spintronic applications. Spintronic devices differ from conventional electronics in that they focus on the intrinsic spin angular momentum of electrons rather than their charge. For example, a “spin current” is one that propagates, not through the transport of charge, but via the transmission of wobbles (spin-axis precession) from one electron to another.
Insulating AFM materials have been shown to be particularly efficient at transporting spin current while blocking the flow of charge, which greatly reduces the power losses associated with moving electrons. One of the most intriguing unanswered questions about AFM materials is whether they can transport spin information coherently—using spin waves with a fixed phase relationship—since this would provide an additional degree of freedom (i.e., the phase) with which to encode information.
Antiferromagnetic spin-current amplifier
Insulating antiferromagnetic NiO thin films have received particular attention recently because they not only transmit spin currents, they can also amplify them. This unique property of NiO has been the subject of intense debate, and it has remained unclear how spin angular momentum can be amplified while passing through the AFM.
To solve this puzzle, a team of researchers focused on the time-varying (ac) component of the spin current, in contrast to previous studies that focused on the time-averaged (dc) spin current. An antiferromagnetic NiO spacer layer was sandwiched between two ferromagnetic layers, which acted as the source (NiFe) and receiver (CoFe) of a gigahertz-frequency spin current, allowing the spin current propagating through the AFM to be quantified. A similar geometry was used in an earlier ALS experiment, but using a nonmagnetic material as the spacer layer.
Probing the flow of spins
At ALS Beamline 4.0.2 and Diamond Light Source Beamline I10, the researchers conducted x-ray ferromagnetic resonance (XFMR) experiments using x-ray magnetic circular dichroism (XMCD) and also x-ray magnetic linear dichroism (XMLD) to explore the dynamic and static magnetic properties of the source/AFM/receiver trilayer system. By using element-specific and time-resolved XFMR (where polarized x-ray pulses probe the magnetization after increasing delays), the spin precession could be measured separately for the source and receiver layers. This unique experimental technique allowed both the amplitude and phase of the ac spin current propagating through the NiO layer to be quantified.
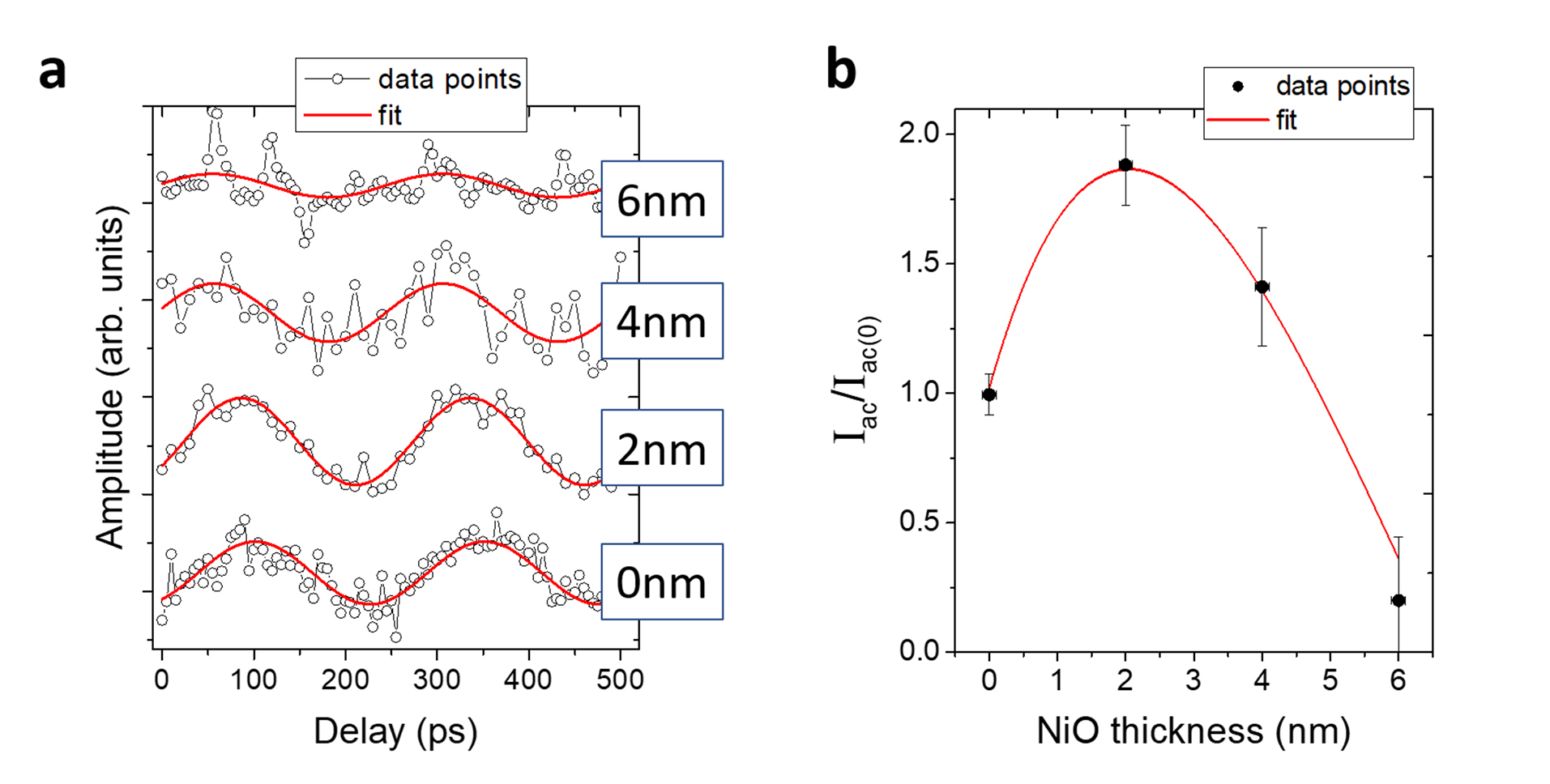
The data demonstrated that, not only is the spin current transmitted coherently, but at a particular NiO thickness (2 nm), the magnitude of the ac spin current was amplified to nearly twice the value it had without the AFM spacer layer. The results are in agreement with a theoretical model based on the excitation of evanescent spin waves: exponentially decaying waves that can “tunnel” through a material boundary. Specifically, the underlying mechanism involves the excitation of two linearly polarized spin waves in the AFM. This enables the transfer of angular momentum between the AFM crystal lattice and the spins, which can lead to amplification of the spin current at certain AFM layer thicknesses. The experimental realization of the amplification of a coherent spin current at the nanoscale and at room temperature advances the use of antiferromagnets toward realistic applications in information processing.
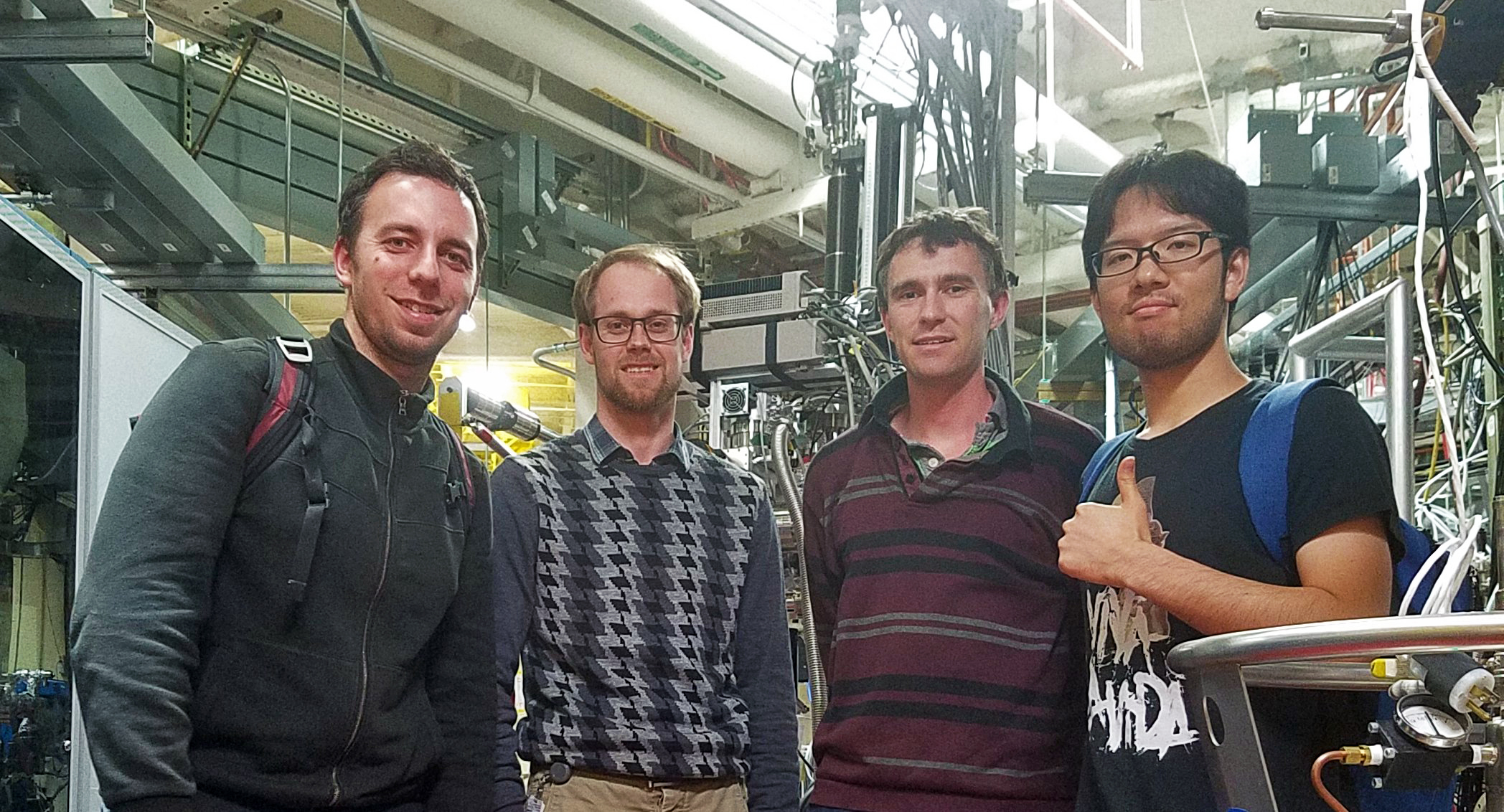
Contacts: Maciej Da̧browski and Robert J. Hicken
Researchers: M. Da̧browski, D.G. Newman, and R.J. Hicken (University of Exeter); T. Nakano (University of Exeter and National Institute of Advanced Industrial Science and Technology, Japan); D.M. Burn, A. Frisk, and G. van der Laan (Diamond Light Source, UK); C. Klewe, P. Shafer, and E. Arenholz (ALS); Q. Li, M. Yang, and Z.Q. Qiu (University of California, Berkeley); and T. Hesjedal (University of Oxford, UK).
Funding: Engineering and Physical Sciences Research Council (UK); U.S. Department of Energy, Office of Science, Basic Energy Sciences program (DOE BES); and Japan Program for the Promotion of Science. Operation of the ALS is supported by DOE BES.
Publication: M. Da̧browski, T. Nakano, D.M. Burn, A. Frisk, D.G. Newman, C. Klewe, Q. Li, M. Yang, P. Shafer, E. Arenholz, T. Hesjedal, G. van der Laan, Z.Q. Qiu, and R.J. Hicken, “Coherent transfer of spin angular momentum by evanescent spin waves within antiferromagnetic NiO,” Phys. Rev. Lett. 124, 217201 (2020), doi: 10.1103/PhysRevLett.124.217201.
ALS SCIENCE HIGHLIGHT #423