Magnetic iron oxides (ferrites) have broad applications in electronics—for example, in communications, sensors, and high-efficiency spintronic devices. These ferrites are complex materials, often containing positively charged magnetic ions (cations) with various charge states and surrounding oxygen geometries (coordination). Because ferrite-based devices are often driven by microwaves, researchers wanted to know how the cations’ magnetic moments (vectors representing each cation’s magnetic field) behave when excited by a microwave field.
“The research community had assumed that these magnetic moments behave uniformly—that they spin around with the same phase,” said Satoru Emori, a physicist at Virginia Tech and principal investigator of this study. “However, the situation might be less clear-cut in complex ferrites, where distinct magnetic cations could interact with their surroundings differently.”
Emori and colleagues studied a thin film of nickel-zinc-aluminum-ferrite (NZAFO), originally developed at the Air Force Research Laboratory. This material exhibits low damping—the spinning magnetic moments experience little “friction”—and contains a diversity of magnetic iron and nickel cations. In addition, NZAFO contains nonmagnetic cations (zinc and aluminum) that dilute the coupling among the magnetic cations, possibly resulting in noticeably different dynamics.
At ALS Beamline 4.0.2, the team conducted x-ray ferromagnetic resonance (XFMR) studies. XFMR combines the elemental, chemical, and coordination sensitivity of x-ray magnetic circular dichroism (XMCD) with the ability to excite magnetic moments with a microwave field. This provides the unique ability to directly investigate the magnetization dynamics of different excited states.
“We were able to push the phase sensitivity of XFMR close to the practical limit and exceeded our own expectations,” said Christoph Klewe, a research scientist at the ALS. “This high precision ended up being critical to confidently answer whether the magnetic moments maintain their alignment during dynamic excitation and to establish a small upper limit for any deviations.”
The results showed that the cations’ magnetic moments stayed tightly in sync and experienced identical damping. The findings validated a long-standing assumption about magnetic oxide dynamics, which was previously difficult to test with enough precision. In the future, the technique may be extended to other kinds of magnetization dynamics, perhaps on even faster time scales.
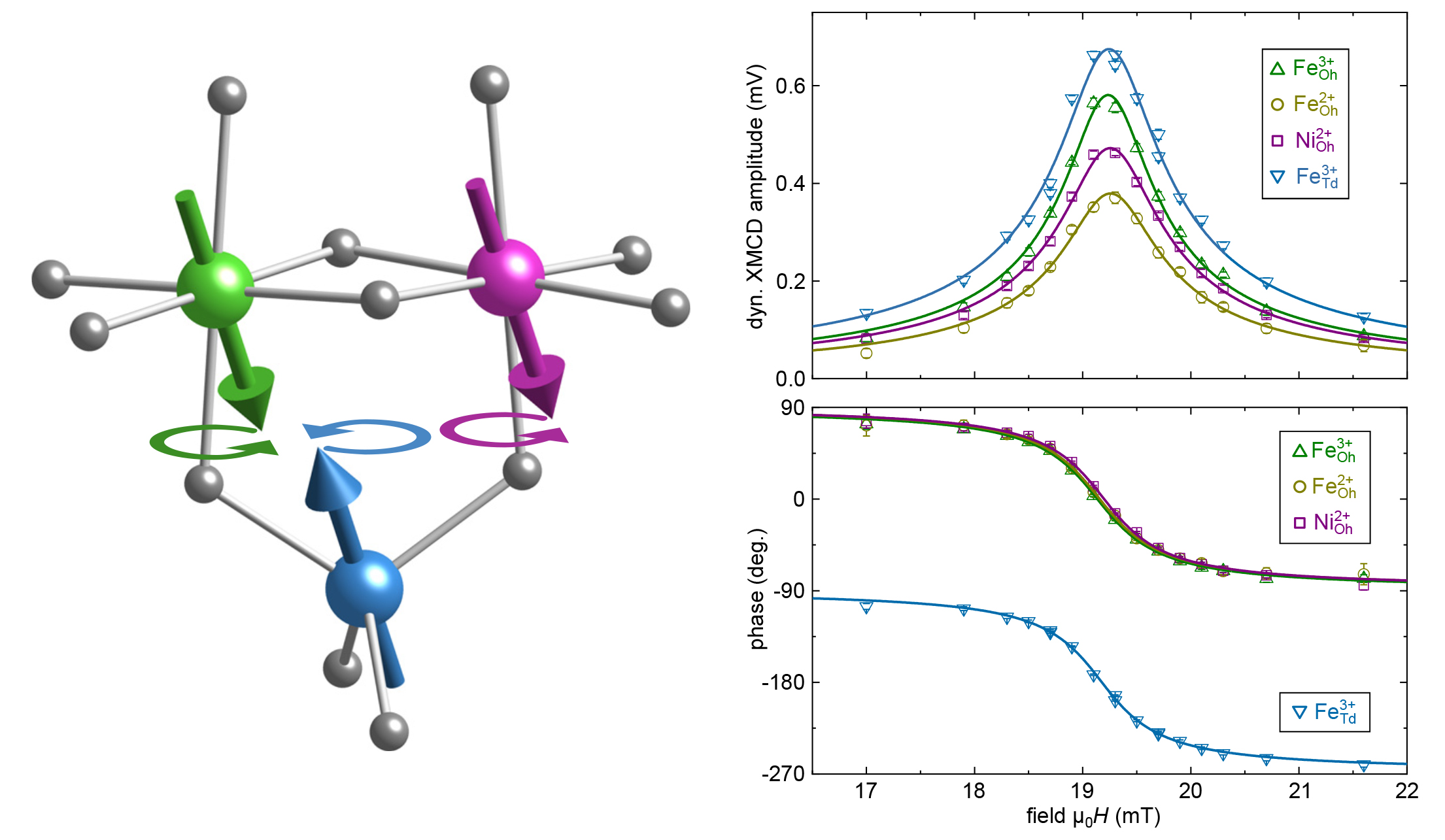
C. Klewe, P. Shafer, J. Shoup, C. Kons, Y. Pogoryelov, R. Knut, B. Gray, H. Jeon, B. Howe, O. Karis, Y. Suzuki, E. Arenholz, D. Arena, and S. Emori, “Observation of Coherently Coupled Cation Spin Dynamics in an Insulating Ferrimagnetic Oxide,” Appl. Phys. Lett. 122, 132401 (2023), doi:10.1063/5.0141869.