Earth’s underground environment can serve as a source of fossil-fuel energy, a containment system for carbon dioxide (CO2), or even a reservoir for the short-term storage of energy from intermittent sources such as wind and sun. Accurate assessment of the safety and profitability of these activities requires a good understanding of how fractures evolve in the subsurface rock matrix. Therefore, researchers have combined time-resolved x-ray microtomography at the ALS with advanced computer simulations to improve models of fracture development in carbonate-rich shale samples. New hardware and software tools able to predict the behavior of fractures in caprock (a generally nonpermeable top layer of rock) can increase confidence in caprock integrity and general storage security.

The role of fractures in controlling the overall hydraulic properties of rocks can be crucial: an intrinsically impermeable rock can become extremely permeable when pervasively fractured. In reactive fluid flow, the fluid affects the physical and chemical characteristics of the surrounding rock, and fractures will evolve over time. Therefore, an understanding of the dynamic evolution of fractures is critical for the assessment and prediction of the fate of fluids and chemicals that are of economic and environmental concern. Relevant applications include enhanced oil recovery and geologic carbon storage, where the injected fluid (CO2) promptly reacts with specific mineral phases (typically carbonates), creating a complex, dynamic system where geochemistry, geomechanics, and hydrology are tightly interconnected.
In this context, a Berkeley-based research team studied, via a coupled experimental and modeling approach, a single fracture in a carbonate-rich shale sample from the Niobrara geological formation in Colorado. The experiment provided the base and the validation datasets for the model; the final goal was to obtain a predictive tool able to overcome experimental limitations.
Researchers from the Energy Geosciences Division of Berkeley Lab developed a state-of-the-art mini flow cell that could be placed into the hard x-ray microtomography beamline at the ALS (Beamline 8.3.2). With this setup, the team was able to monitor, in real time, the evolution of the 3D microstructure of the rock sample as CO2-saturated water flowed through the fracture at realistic subsurface pressures and temperatures.
The sequences of tomographic images obtained show the evolution of the fracture aperture and the weathering processes involving the rock’s microstructure. The highly reactive minerals (calcite) promptly dissolve along preferential flowpaths, and the surface of the fracture becomes weathered, gradually exposing a porous and poorly coherent layer of unreactive minerals (clays, quartz, and feldspars) on the surface. As the cementing mineral (calcite) dissolves, the exposed, less reactive, fine minerals eventually disaggregate and detach from the fracture surface. This mechanism results in a constant enlargement of the aperture along the flowpaths. The effect of this erosion is usually not considered in conventional reactive-transport models, but as the experiment suggests, this could lead to underestimating the potential leakage from fractures.
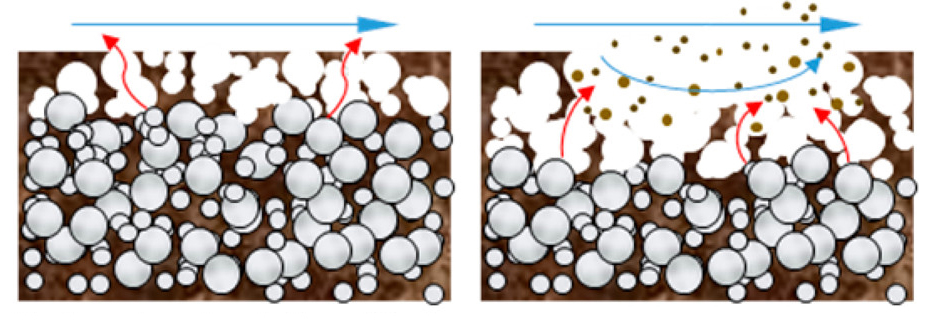
In light of the experimental observations, an erosion module was added to an existing reactive-transport model, to account for the detachment of the altered layer. The erosion rate of the altered layer was formulated as a function of its thickness, which is a measure of the extent of dissolution of the fast-reacting cementing mineral. The parameters needed for this calculation can be well constrained by experimental measurements, such as the thickness of the altered layer taken from the microtomography images. The model results capture the temporal evolution of the geochemical reactions and fracture morphology.
This predictive model incorporates complex dynamics that arise from heterogeneous mineral compositions, e.g. the erosion of nonreactive matrix triggered by the dissolution of the fast-reacting mineral, and provides a valuable tool for investigating fracture evolution in mineralogically complex systems under conditions beyond those of current experimental capabilities.
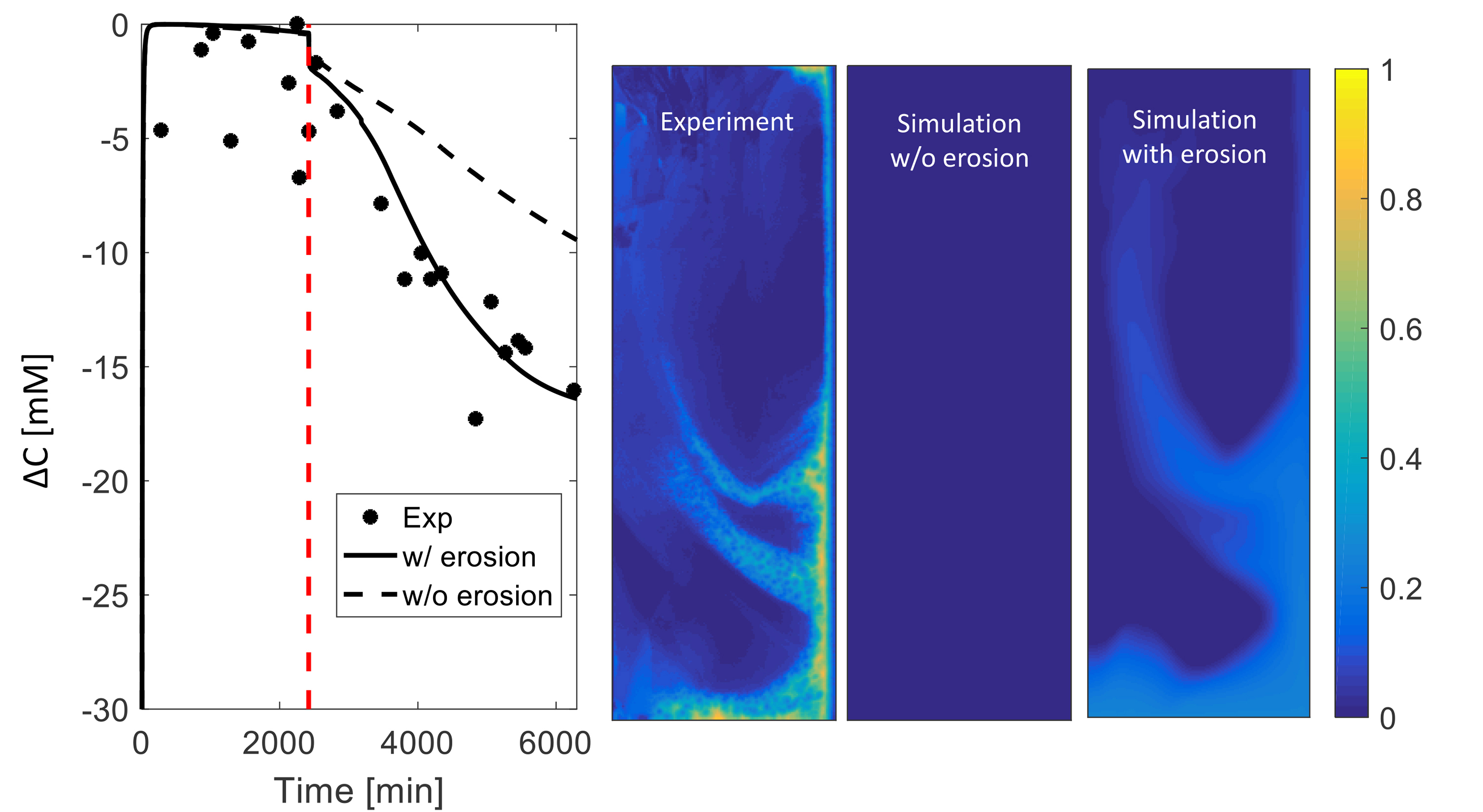
Contacts: Hang Deng and Marco Voltolini
Research conducted by: H. Deng, M. Voltolini, S. Molins, C. Steefel, J. Ajo-Franklin, and L. Yang (Berkeley Lab) and D. DePaolo (Berkeley Lab and UC Berkeley).
Research funding: U.S. Department of Energy, Office of Science, Basic Energy Sciences Program (DOE BES). Operation of the ALS is supported by DOE BES.
Publication about this research: H. Deng, M. Voltolini, S. Molins, C. Steefel, D. DePaolo, J. Ajo-Franklin, and L. Yang, “Alteration and Erosion of Rock Matrix Bordering a Carbonate-Rich Shale Fracture,” Environ. Sci. Technol. 51, 8861 (2017). doi: 10.1021/acs.est.7b02063
ALS SCIENCE HIGHLIGHT #363