HP Labs researchers have tackled a decades-old mystery relying on powerful ALS microscopy techniques to better understand the fourth basic circuit element: the memristor. The memristor (short for “memory resistor”) joins the other passive elements—the capacitor, the resistor, and the inductor—to create a device with the ability to “remember” changes even when it loses power. Commercial development based on memristors offers the promise of computing systems with highly advanced energy efficiency and memory retention. Memristor-based memory could be a strong competitor for current flash memory.
“The basic idea is that if you run current through a device you’ll not only measure a certain resistance, but that same current—if strong enough—will also be transforming that resistance,” says John Paul Strachan (at left), Research Scientist at HP Labs whose team develops new electronic and optical devices and applications. “This has clear cut applications in memory – you’re able to store that state and then read it out and rewrite it.”
The concept of the memristor had been theoretically developed in 1971, when UC Berkeley professor Leon Chua published a paper predicting that a memristor would have certain mathematical properties and be useful in many ways. At the same time and even earlier, many researchers had observed resistance switching behavior in a number of materials and were working to produce a microphysical description to explain how and why the resistance was changing so dramatically. But much of this research had been done without advanced techniques to probe the devices, and without connecting this observed behavior to Chua’s memristor.
HP Labs researchers were able in 2006 to show that the devices many earlier researchers had been studying – basically just metal-insulator-metal sandwiches—were in fact physical realizations of the memristor. The devices themselves can be several square micrometers in area or much smaller, with the insulating material just a few nanometers thick and buried between two contacting electrodes.
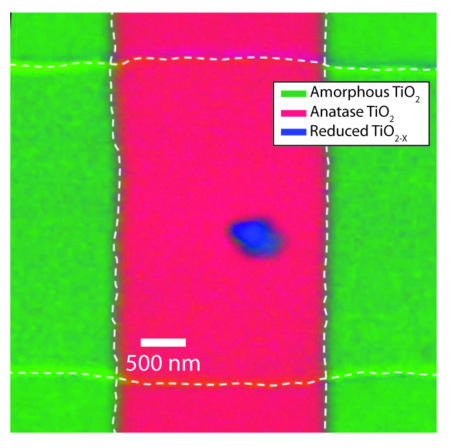
“With the evolution of synchrotrons and the development of powerful microscopy techniques, we’ve been able to probe these devices with tens of nanometers resolution and get all sorts of information about the local chemistry, the structure and the elemental mapping,” says Strachan. “The techniques now available to us at the ALS are relatively recent developments and they’ve allowed us to revisit this very old research topic, rule out a whole lot of theories that had been suggested, and come a lot closer to understanding the basic physics of how these devices operate.”
Soft x-ray scanning transmission x-ray microscopy (STXM) capabilities available at ALS Beamlines 5.3.2 and 11.0.2 are uniquely matched to memristor research because the x-rays interact just weakly enough that researchers are able to probe beneath the electrodes and just strongly enough to get plenty of signal from a thin film, says Strachan. “On top of that, we get resolution of 30 nanometers and chemical and structural information about what’s going on in our devices,” he says.
Strachan started working on memristors in 2008 and by the end of that year it was pretty clear that researchers could learn a lot from the techniques available at the ALS. In 2009 his group published their first paper based on research at the ALS.
Strachan’s ALS research identified an interesting compound in memristor devices. Using STXM to examine one of their titanium dioxide (TiO2) memristors after it had been run for a number of cycles, he found that there was a region inside the device where oxygen vacancies had condensed and ordered to form the compound Ti4O7.
“This turned out to be a really interesting discovery. It was a combination of electrochemistry to create these oxygen vacancies and heating to condense them into the local structure of a Mágneli phase (Ti4O7),” says Strachan. “Knowing that we had formed this Mágneli phase in the device, we started learning some of the key physical ingredients to switching memristors – namely, electrochemistry and thermal processes.”
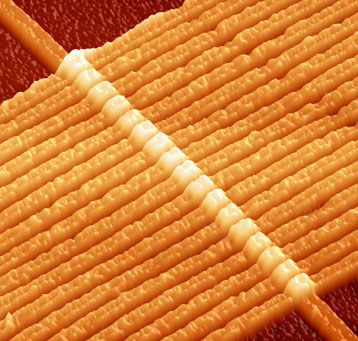
This Mágneli phase was also interesting because it undergoes an insulator-to-metal transition when heated up. Inserting this material into an electronic device and running current through it leads to a striking negative differential resistance as that current heats it up past its transition temperature from insulator to metal. This discovery was one of the insights in the development of the neuristor, which is a device that reproduces some properties of neurons.
Strachan is now using the STXM facilities at the ALS to understand more about neuristors, and would like to thank ALS scientist David Kilcoyne (Beamline 5.3.2) and Molecular Foundry scientist Shaul Aloni for their help on this research. “Both memristor and neuristor research are being pushed to the next level with the techniques and capabilities available at the ALS,” says Strachan.