Studying and identifying molecules at the mesoscale has always been challenging—even the best microscopes and spectrometers have difficulty simultaneously identifying and spatially resolving this realm of matter, which ranges from about 10 to 1000 nanometers in size. But ALS researchers recently developed a broadband imaging technique that looks inside the mesoscale realm with unprecedented sensitivity and range. The new technique, called Synchrotron Infrared Nano-Spectroscopy (SINS), will enable in-depth study of complex molecular systems, including liquid batteries, living cells, novel electronic materials, and stardust. SINS gives researchers the power of full broadband infrared spectroscopy at 100 to 1000 times smaller scale than previously possible, an unprecedented level of resolution.
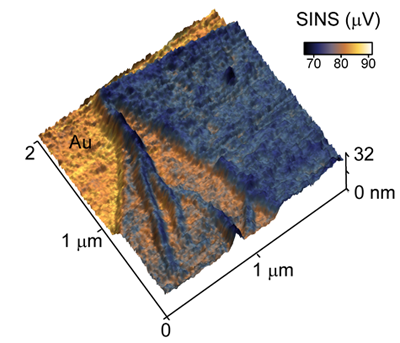
By combining atomic force microscopy with infrared synchrotron light, ALS researchers from Berkeley Lab and the University of Colorado have improved the spatial resolution of infrared spectroscopy by orders of magnitude, while simultaneously covering its full spectroscopic range, enabling the investigation of variety of nanoscale, mesoscale, and surface phenomena that were previously difficult to study. The researchers have demonstrated SINS’ ability to capture broadband spectroscopic data over a variety of samples, including a semiconductor-insulator system, a mollusk shell, proteins, and a peptoid nanosheet.
SINS combines two pre-existing infrared technologies: a newer technique called infrared scattering-scanning near-field optical microscopy (IR s-SNOM) and an older tried-and-true technique called Fourier transform infrared spectroscopy (FTIR). A melding of these two tools, combined with the intense infrared light of the ALS, gives the researchers the ability to identify and study clusters of molecules sized as small as 20 to 40 nanometers.
The new approach overcomes long-standing barriers with pre-existing microscopy techniques that often involve demanding technical and sample preparation requirements. Infrared spectroscopy uses low-energy light, is minimally invasive, and is applicable under ambient conditions, making it an excellent tool for chemical and molecular identifications in systems that are static as well as those that are living and dynamic. The technique works by shining low-energy infrared light onto a molecular sample. Molecules can be thought of as systems of balls (atoms) and springs (bonds between atoms) that vibrate with characteristic wiggles; they absorb infrared radiation at frequencies that correspond to their natural vibrating modes. The output from this absorption is a spectrum, often called a fingerprint, that shows distinctive peaks and dips, depending on the bonds and atoms present in the sample.
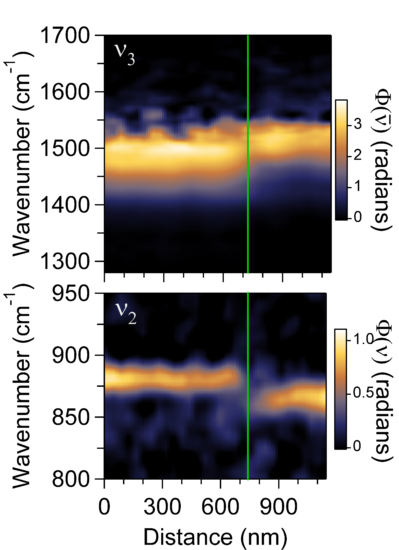
But infrared spectroscopy has its challenges too. While it works well for bulk samples, traditional infrared spectroscopy can’t resolve molecular composition below about 10,000 nanometers. The major hurdle is the diffraction limit of light, which is the fundamental barrier that determines the smallest focus spot of light and is particularly troublesome for the large wavelengths of infrared light. In recent years, though, the diffraction limit has been overcome by a technique called scattering-scanning near-field optical microscopy, or s-SNOM, which involves shining light onto a metallic tip. The tip acts as an antenna for the light, directing it to a tiny region at its apex just tens of nanometers wide.
This trick is what’s used in IR s-SNOM, where infrared light is coupled to a metallic tip. The challenge with IR s-SNOM, however, is that researchers have been relying on infrared light produced by lasers. Lasers emit a large number of photons needed for the technique, but because they operate in a narrow wavelength band, they can only probe a narrow range of molecular vibrations. In other words, laser light simply can’t give you the flexibility to explore a spectrum of mixed molecules.
The capabilities of the ALS helped the researchers overcome the laser limitation. Because the ALS produces broadband infrared light with a high photon count that can be focused to the diffraction limit, the researchers were able to couple the synchrotron light to a metallic tip with an apex of about 20 nanometers and produce infrared spectra with a modified FTIR instrument.
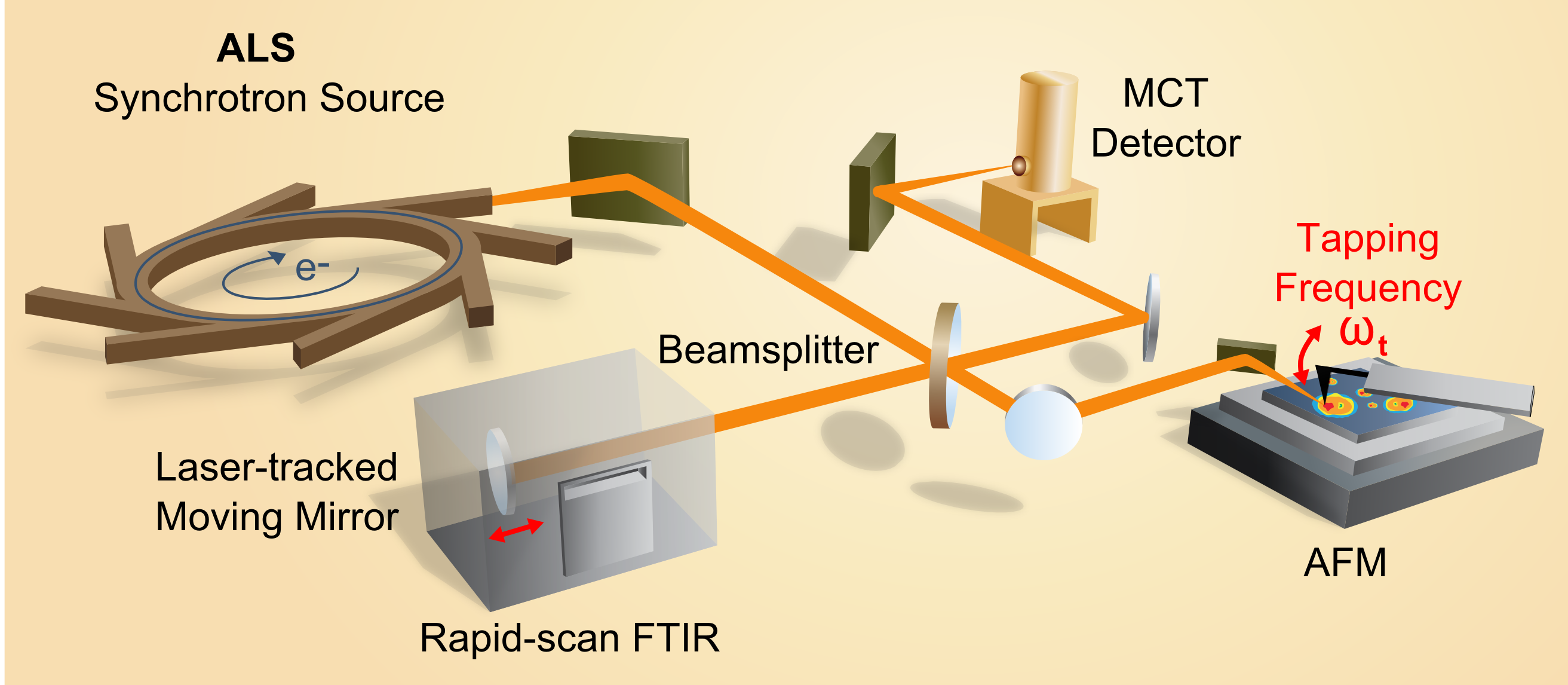
Contact: Michael C. Martin
Research conducted by: H.A. Bechtel and M.C. Martin (ALS); and E.A. Muller, R.L. Olmon, and M.B. Raschke (Univ. of Colorado, Boulder, and NIST).
Research funding: Operation of the ALS is supported by the U.S. Department of Energy, Office of Basic Energy Sciences.
Publication about this research: H.A. Bechtel, E.A. Muller, R.L. Olmon, M.C. Martin, and M. B. Raschke, “Ultrabroadband infrared nanospectroscopic imaging,” PNAS 111, 7191 (2014).
ALS SCIENCE HIGHLIGHT #291