SCIENTIFIC ACHIEVEMENT
X-ray scattering experiments at the Advanced Light Source (ALS) helped reveal how nanosized crystals (“quantum dots”) self-assemble and fuse to form “supercrystals” with potentially useful electronic properties.
SIGNIFICANCE AND IMPACT
The findings provide new insight into the fabrication of high-performance, low-cost electronic materials for photovoltaic and photon-sensing applications.
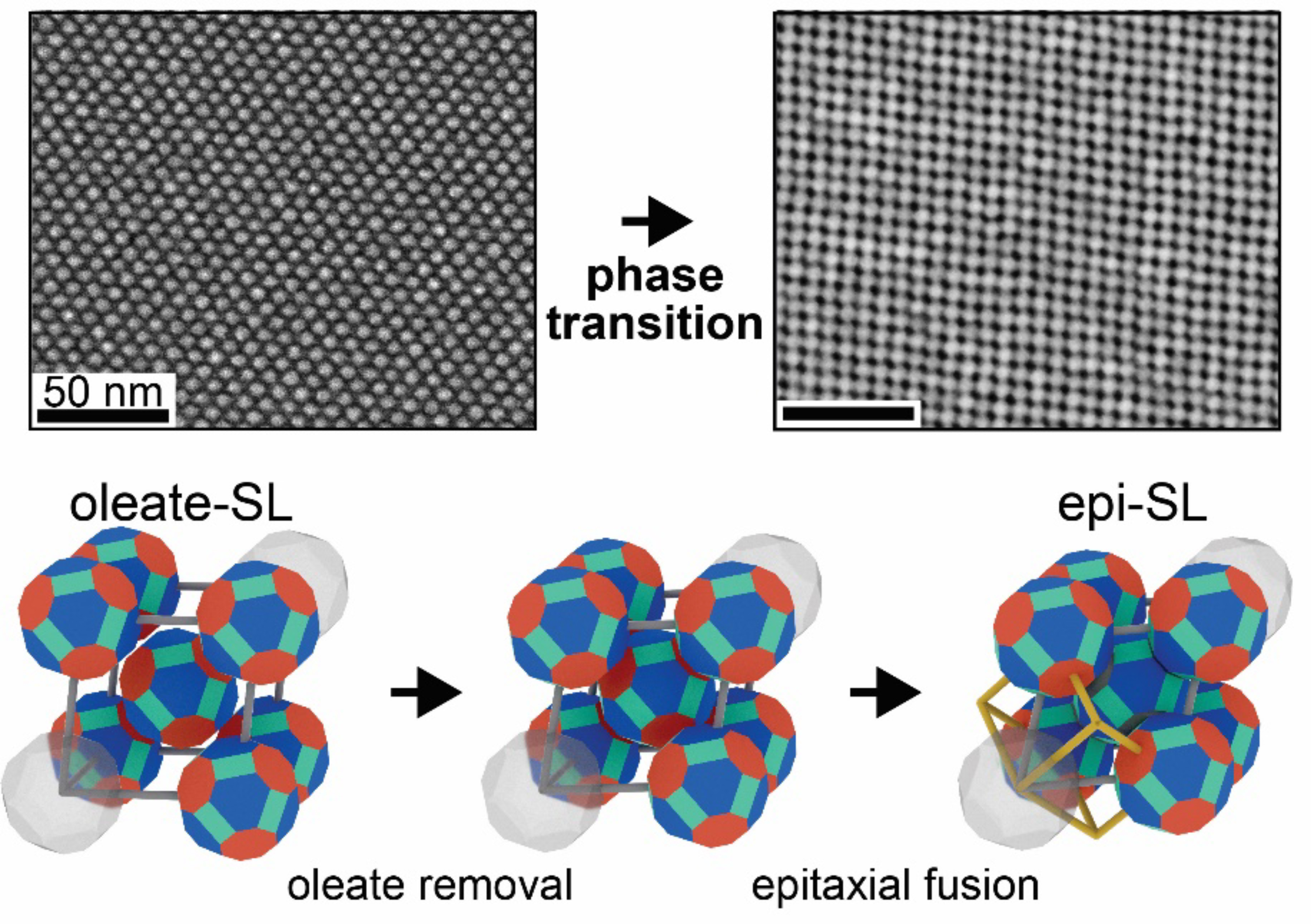
A playground for mesoscale science
Quantum dots are tiny crystals, typically a few nanometers in diameter, that can be cost-effectively synthesized in large batches with fine control over composition, size, and shape. Their small size means that their electronic and optical properties are subject to quantum-mechanical effects. Together, these factors make quantum dots useful building blocks for highly tunable mesoscale materials—those with properties that bridge the gap between bulk solids and individual atoms or molecules.
Films made up of quantum dots show promise for use in solar cells and other light-sensing applications. However, they usually suffer from large amounts of disorder, hindering the efficient movement of charge through the material. In this study, x-ray scattering and electron microscopy were combined to reveal the formation mechanisms of three-dimensional arrays (superlattices) of fused quantum dots. The results bring us a step closer to the rational fabrication of novel materials with emergent mesoscale properties.
Superlattices, before and after fusion
The researchers studied lead selenide (PbSe) quantum dots arranged into superlattices. Initially, the quantum dots are capped by a thin protective layer of organic molecules (oleate). Chemical removal of the oleate triggers the physical (epitaxial) fusion of the quantum dots. Epitaxially fused quantum dot superlattices are predicted to exhibit bulk-like electronic bands, indicating the presence of “delocalized” electrons free to move about the material, thus combining the size-tunable optical properties of quantum dots with the efficient charge-transport properties of bulk semiconductors.
To better understand the details of quantum dot fusion, the researchers determined the structures of three-dimensional superlattices, before and after quantum dot fusion, using electron microscopy at UC Irvine and grazing-incidence small-angle x-ray scattering (GISAXS) at ALS Beamline 7.3.3. The beamline’s high x-ray fluence, narrow energy dispersion, and versatile sample-mounting options were critical for this work. The variable sample-to-detector distance within the “flight tube” allowed the optimization of data collection for the best possible results.
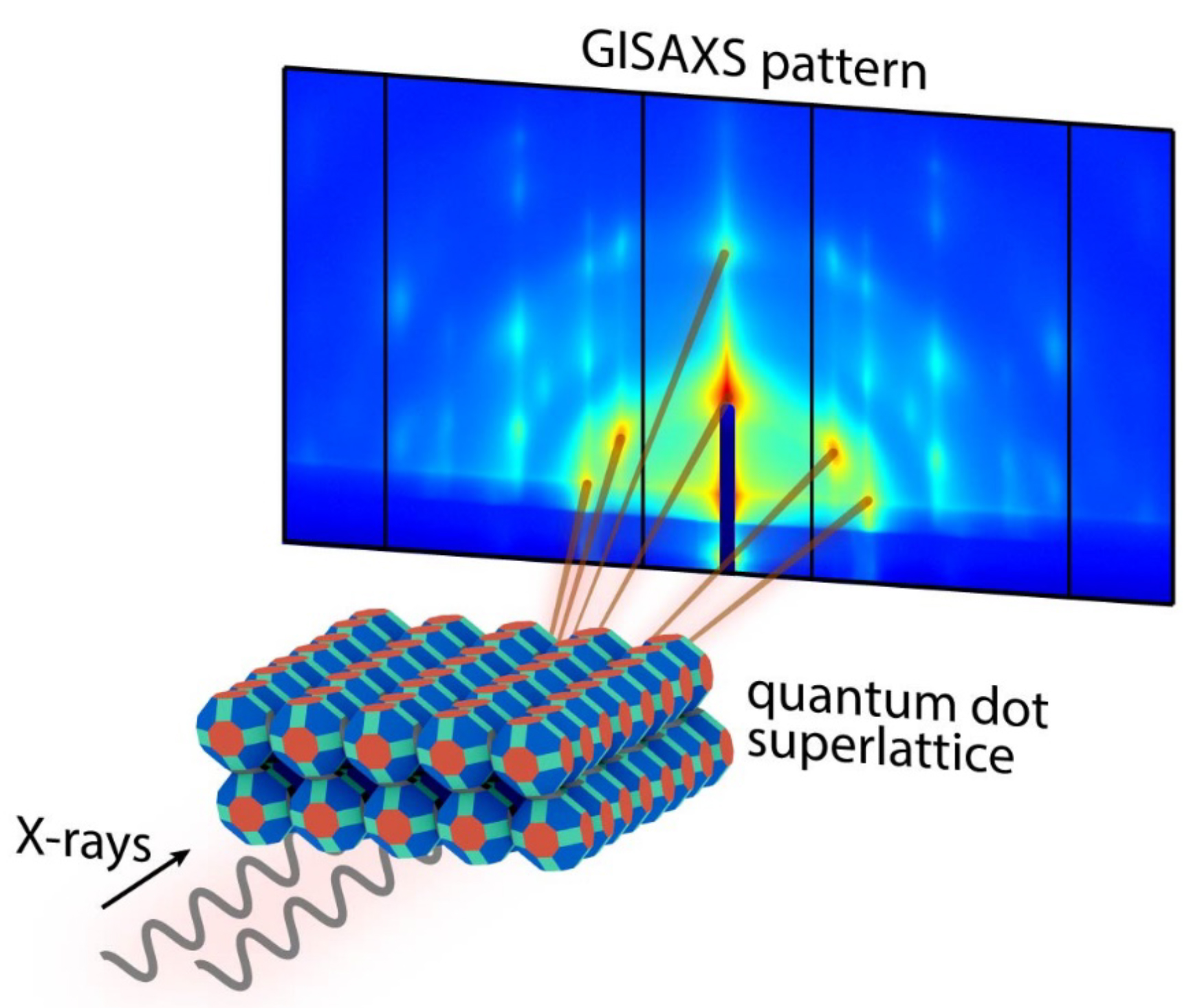
The transformation pathway
The data revealed that the oleate-capped superlattice adopts a rhombohedrally distorted body-centered cubic structure (imagine stretching apart two opposing corners of a cube). The epitaxially fused superlattice adopts a rhombohedrally distorted, simple cubic unit cell structure. This transformation occurs through concerted rotation (about 10°) and translation (about 1.6 nm) of each quantum dot relative to its neighbor. The small rotation was particularly surprising, since two-dimensional superlattices were previously shown to require concerted 45˚ rotations. Reducing the required rotation should increase the fidelity of the transformation.
Another surprising finding was the mechanism by which the chemical trigger (ethylenediamine) initiates the conversion process. The researchers discovered a critical intermediary step in which the ethylenediamine deprotonates the reaction solvent (ethylene glycol), which in turn exchanges for oleate and triggers the phase transition. This insight should enable finer control of superlattice surface chemistry and the structural transformation kinetics.
Future efforts will focus on the details of the crystalline necks that adjoin neighboring quantum dots. For example, what atomistic defects are present and how might they affect the emergence of bulk-like transport? These fine structural details are challenging to extract—particularly for three-dimensional superlattices—but are critically important for band-like transport.
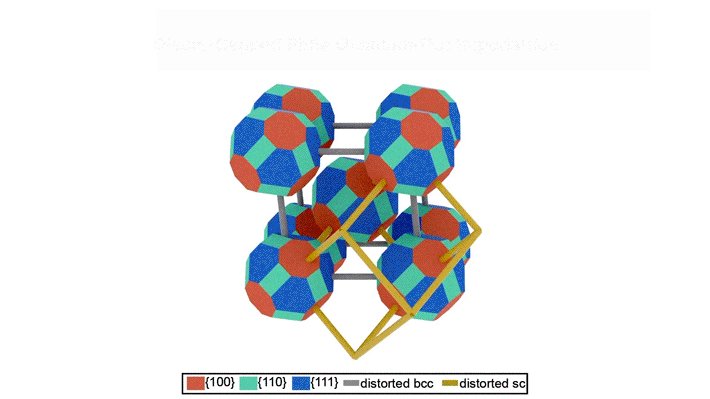
Contacts: Alex Abelson and Matt Law
Researchers: A. Abelson, C. Qian, T. Salk, Z. Luan, K. Fu, J.-G. Zheng, J.L. Wardini, and M. Law (Univ. of California, Irvine).
Funding: Univ. of California Office of the President and National Science Foundation. Operation of the ALS is supported by the U.S. Department of Energy, Office of Science, Basic Energy Sciences Program.
Publication: A. Abelson, C. Qian, T. Salk, Z. Luan, K. Fu, J.-G. Zheng, J.L. Wardini, and M. Law, “Collective topo-epitaxy in the self-assembly of a 3D quantum dot superlattice,” Nat. Mater. 19, 49 (2020), doi:10.1038/s41563-019-0485-2.
ALS SCIENCE HIGHLIGHT #410