SCIENTIFIC ACHIEVEMENT
Researchers directly observed the formation of highly oxygenated molecules—the elusive intermediate products of auto-oxidation reactions relevant to combustion and atmospheric chemistry.
SIGNIFICANCE AND IMPACT
A better understanding of auto-oxidation reaction mechanisms could lead to better engines, less air pollution, and improved climate models.
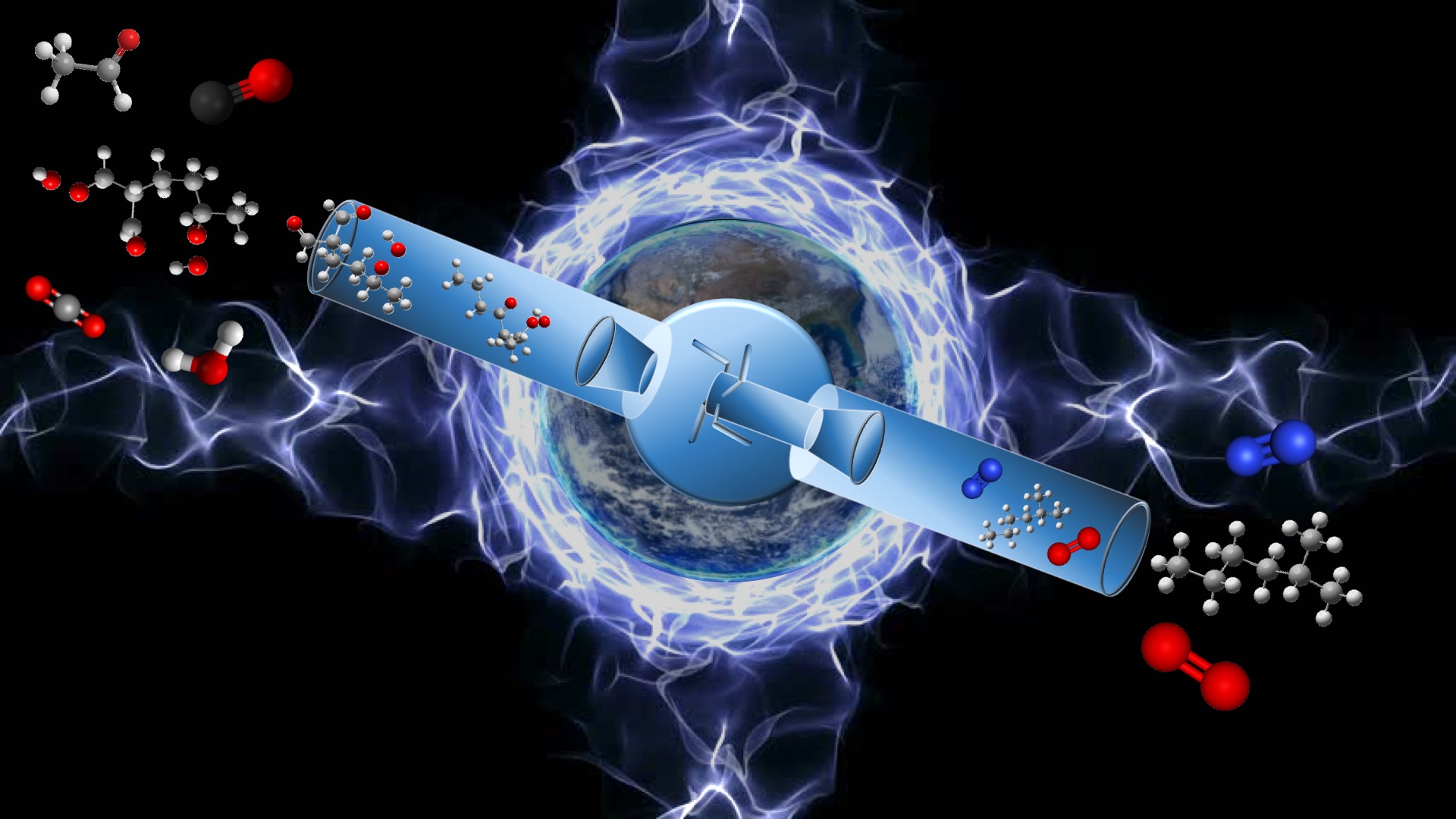
Highly oxygenated molecules: vital but ephemeral
Oxygen plays a key role in chemical reactions that produce pollutants in the atmosphere and that fuel combustion engines. In these reactions, auto-oxidation (spontaneous oxidation in air or in the presence of oxygen) produces highly oxygenated molecules.
For example, volatile organic compounds (VOCs)—gas molecules emitted from vehicles, factories, and power plants (as well as living plants)—undergo a sequence of auto-oxidation reactions with the surrounding air, forming highly oxygenated molecules that contribute to air pollution, affecting health and climate. The same highly oxygenated molecules are also produced during the ignition of fuels in advanced combustion engines; controlling their formation and destruction processes can help to develop higher-efficiency engines.
However, because these highly oxygenated molecules are very reactive and decompose quickly, determining their identities has been difficult.
“Freezing” the reaction at ALS Beamline 9.0
In this work, researchers investigated 15 VOCs representing species present in tropospheric (lower atmosphere) and fuel/engine environments. Auto-oxidation of the VOCs occurred in a jet-stirred reactor: gases enter at one end, undergo turbulent mixing by jets in the middle, and exit the opposite end as reaction products. A steep drop in pressure at the stage of molecular-beam sampling prevents further interactions between molecules. This “freezes” the chemistry, enabling the detection of highly reactive species.
The reaction products were photoionized by vacuum-ultraviolet (VUV) light from the ALS’s Chemical Dynamics Beamline (9.0.2). The resulting photoions were analyzed via mass spectrometry to identify the parent molecules. Because synchrotron VUV light can be tuned to near-threshold ionization energies (“soft” ionization), fragmentation from excess energy was minimized, simplifying data interpretation.
Further experiments sharpen the focus
To enhance the mass spectrometry data, the researchers employed isotope labeling techniques. One form of this involves replacing 16O2 with 18O2 as the oxidizer. By noting how this changes the mass-to-charge ratio (m/z) in the mass spectrometry signal, it’s possible to obtain unambiguous elemental compositions of the highly oxygenated intermediates. Another trick is to exchange hydrogen (H) for deuterium (D) by adding D2O to the reaction system. This helps identify the number of hydroperoxy (–OOH) functional groups in an intermediate. Hydroperoxides are important in controlling hydrocarbon reactivity during auto-oxidation. Finally, photoionization efficiency (PIE) curves were recorded; these provide precise information about ionization energies and can be used in conjunction with m/z to differentiate species of equal or near-equal masses.

Elucidating the elusive
Taken together, the results of this comprehensive study elucidate the underlying kinetics and structural characteristics of auto-oxidation processes. In particular, the results suggest a generalized reaction mechanism in which at least three stages of sequential oxygen attachments can occur, in contrast to current models of combustion chemistry that assume just one or two. When these pathways are included in models, they significantly alter simulation results. Updating the models will allow more accurate simulations of fuel combustion and potentially improve the performance of real engines.
The researchers are currently working with atmospheric scientists to develop models for atmospheric aerosol formation via VOC auto-oxidation, which could significantly improve simulations predicting air pollution and global temperature.
Contacts: Zhandong Wang and S. Mani Sarathy
Researchers: Z. Wang, B. Chen, S.Y. Mohamed, H. Wang, S. Sioud, M.A. Raji, and S.M. Sarathy (King Abdullah University of Science and Technology, Saudi Arabia); D.M. Popolan-Vaida (Univ. of California, Berkeley; Berkeley Lab; University of Central Florida); K. Moshammer (Sandia National Laboratories, Livermore; Physikalisch-Technische Bundesanstalt, Germany); K. Kohse-Höinghaus (Bielefeld University, Germany); N. Hansen (Sandial National Laboratories, Livermore); P. Dagaut (National Center for Scientific Research, France); and S.R. Leone (University of California, Berkeley; Berkeley Lab).
Funding: King Abdullah University of Science and Technology; Saudi Aramco; U.S. Department of Energy, Office of Science, Basic Energy Sciences Program (DOE BES); German Research Foundation; and European Research Council. Operation of the ALS is supported by DOE BES.
Publication: Z. Wang, D.M. Popolan-Vaida, B. Chen, K. Moshammer, S.Y. Mohamed, H. Wang, S. Sioud, M.A. Raji, K. Kohse-Höinghaus, N. Hansen, P. Dagaut, S.R. Leone, and S.M. Sarathy, “Unraveling the structure and chemical mechanisms of highly oxygenated intermediates in oxidation of organic compounds,” PNAS 114, 13102 (2017), doi: 10.1073/pnas.1707564114.
Adapted in part from the King Abdullah University of Science and Technology (KAUST) press release, “Something in the Air.”
ALS SCIENCE HIGHLIGHT #369