SCIENTIFIC ACHIEVEMENT
Using the Advanced Light Source (ALS) and other synchrotron facilities, researchers discovered that electrons in a high-temperature superconductor can exhibit a new type of collective behavior that is more “glassy” (disordered) than expected.
SIGNIFICANCE AND IMPACT
The study provides valuable insight into the nature of collective electron behaviors and how they relate to high-temperature superconductivity.
Superconductivity and charge order
At extremely low temperatures, superconductors conduct electricity without resistance, a characteristic that’s already being used in cryogenically cooled power lines and quantum-computer prototypes. To apply this characteristic more widely, however, it’s necessary to raise the temperature at which materials become superconducting. Unfortunately, the exact mechanism by which this happens remains unclear.
Recently, scientists found that electrons in cuprate superconductors can self-organize into charge-density waves—periodic modulations in electron density that hinder the flow of electrons. As this effect is antagonistic to superconductivity, tremendous effort has been devoted to fully characterizing this charge-order phase and its interplay with high-temperature superconductivity.
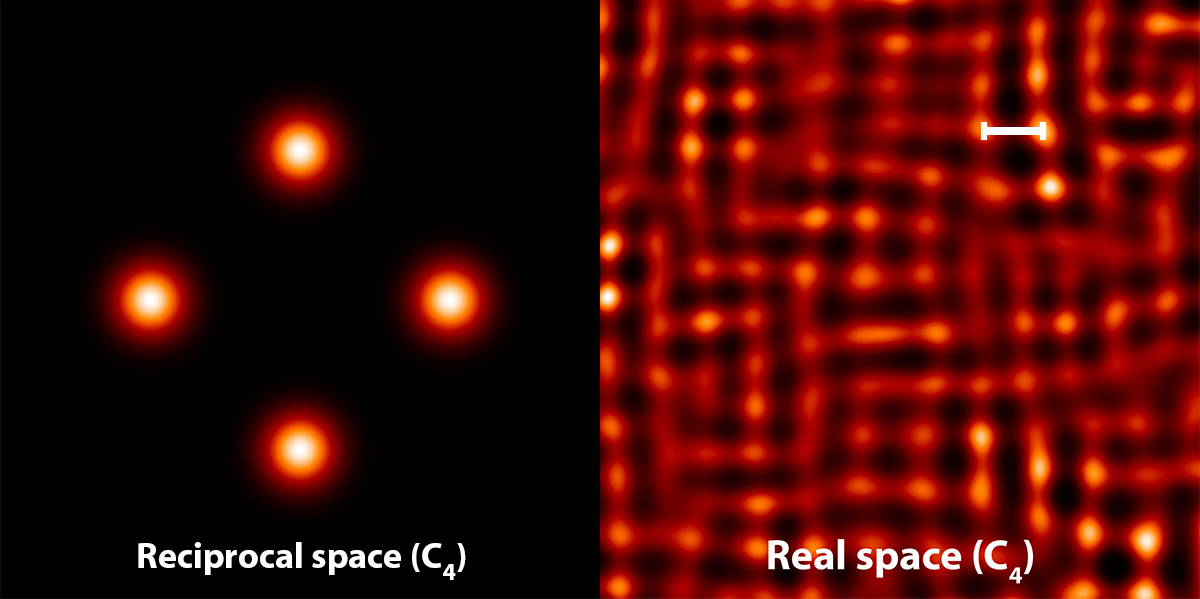
Electron-doped cuprates
Cuprate materials doped with electrons are particularly interesting because their superconducting phases can coexist with antiferromagnetic and charge-order phases. In this work, researchers studied thin films of two electron-doped cuprate superconductors: neodymium copper oxide (Nd2CuO4) and praseodymium copper oxide (Pr2CuO4).
Although previous studies found that charge-density waves align parallel to the copper–oxide bonds in cuprate materials, the situation can be more complex in the presence of the strong electron–electron interactions found in superconductors. In this work, the researchers broadened the investigation of charge order by performing diffraction experiments along multiple directions.
Crystallography of electrons
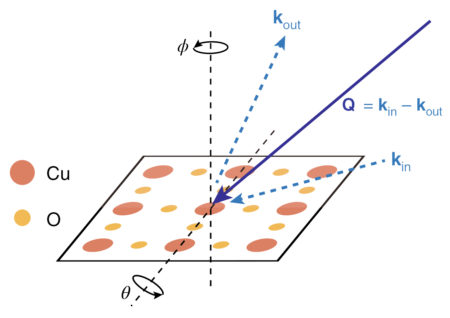
Resonant soft x-ray diffraction (RSXD) is a recently developed diffraction technique that enables crystallography on periodic structures formed by electrons, rather than exclusively on the atomic lattice, as in conventional x-ray diffraction. However, because the scattering strength of charge-density waves in cuprates is very low, the researchers must maximize the small signal by tuning the photons to the appropriate resonances (in this case, the copper L3-edge and oxygen K-edge). Therefore, high energy resolution and precise energy tunability of the light source are essential. Moreover, since RSXD is a photon-hungry technique, a high flux of incident x-rays is also required. The researchers therefore brought their samples to ALS Beamline 4.0.2, as well as to beamlines at the Berlin Electron Storage Ring (BESSY II) and the Canadian Light Source, which offer the requisite tunability, photon energy range, photon flux, temperature range, detectors, and scattering geometries required for successful detection of charge-density waves.
A new phenomenon
When charge order is aligned to the copper–oxygen bond directions, the data in reciprocal space displays a fourfold symmetry. In real space, this corresponds to a bidirectional modulation in the charge density (i.e., a checkerboard pattern). However, the data revealed something completely different: in samples with low electron doping, an electron wave was detected propagating in all directions. In real space, this corresponds to a new type of electronic ordering, where electrons form a periodic array but without any orientational preference. The tendency to form electronic ripples in all directions is indicative of an amorphous glass of electrons (a “Wigner glass”).
Calculations revealed that this behavior arises from the strong interplay between antiferromagnetism and charge order. Specifically, in the low-doping limit, antiferromagnetism coexists with charge order and strongly influences the symmetry of the latter. Correspondingly, in the high-doping limit, where antiferromagnetism disappears, the charge-density waves revert to the more familiar checkerboard pattern. The results will challenge experts to rethink their understanding of charge order and its implications for the origins of high-temperature superconductivity.
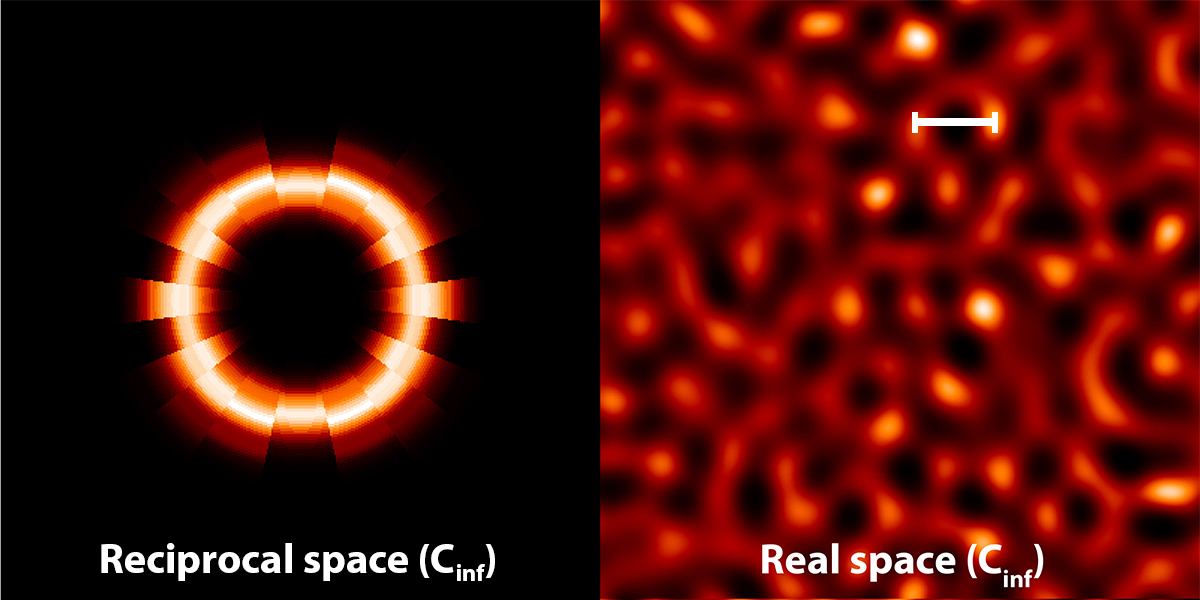
Contacts: Mingu Kang, Riccardo Comin
Researchers: M. Kang, J. Pelliciari, and R. Comin (Massachusetts Institute of Technology); A. Frano (UC Berkeley and UC San Diego); N. Breznay, M. Chen, K. Zhang, A. Ruiz, Z. Hao, S. Lewin, and J. Analytis (UC Berkeley); E. Schierle and E. Weschke (Helmholtz Zentrum Berlin für Materialien und Energie, Germany); R. Sutarto and F. He (Canadian Light Source); P. Shafer and E. Arenholz (ALS); Y. Krockenberger and H. Yamamoto (NTT Basic Research Laboratories, Japan); and T. Das (Indian Institute of Science, India).
Funding: National Science Foundation, Canada Foundation for Innovation, Natural Sciences and Engineering Research Council of Canada, University of Saskatchewan, Government of Saskatchewan, Western Economic Diversification Canada, National Research Council Canada, Canada Institutes of Health Research, Samsung Foundation of Culture, Swiss National Science Foundation, Gordon and Betty Moore Foundation, Infosys Science Foundation, and U.S. Department of Energy, Office of Science, Basic Energy Sciences Program (DOE BES). Operation of the ALS is supported by DOE BES.
Publication: M. Kang, J. Pelliciari, A. Frano, N. Breznay, E. Schierle, E. Weschke, R. Sutarto, F. He, P. Shafer, E. Arenholz, M. Chen, K. Zhang, A. Ruiz, Z. Hao, S. Lewin, J. Analytis, Y. Krockenberger, H. Yamamoto, T. Das, and R. Comin, “Evolution of charge order topology across a magnetic phase transition in cuprate superconductors,” Nat. Phys. 15, 335 (2019), doi:10.1038/s41567-018-0401-8.
ALS SCIENCE HIGHLIGHT #395