SCIENTIFIC ACHIEVEMENT
Using the Advanced Light Source (ALS), researchers discovered a liquid-like layer of electrons that floats on the surface of an unusual crystal and appears to undergo a phase transition upon doping.
SIGNIFICANCE AND IMPACT
The system is an ideal platform for studying exotic phenomena involving electrons (e.g. superconductivity) without complications arising from other types of interactions.
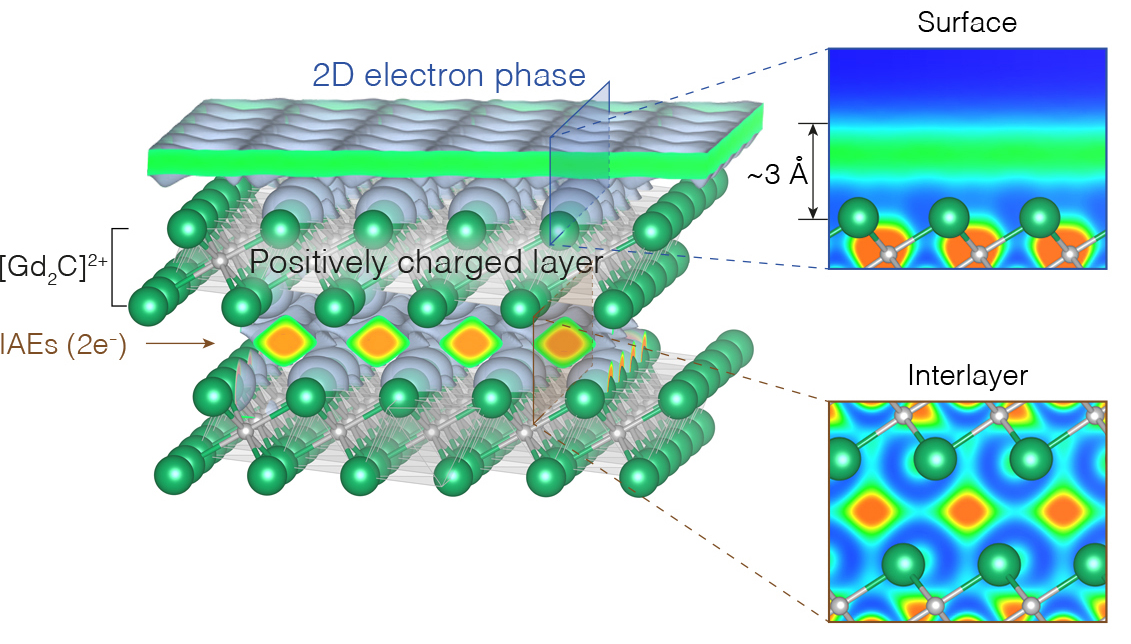
Demand for a “pure” electron system
Like atoms and molecules, electrons can also form gases, liquids, and solids. These electron phases are foundational in physics, chemistry, and materials science. “Pure” electron systems not only shed light on electron–electron interactions, they can also help sort out the roles that other, competing interactions might play in quantum materials.
For example, strongly correlated electron systems exhibit complex behaviors owing to their many interacting variables (lattice, charge, orbit, and spin). While this complexity leads to rich physics, it also makes analysis difficult. Tackling questions about complex phenomena by starting with pure electron systems could unlock answers to longstanding puzzles like unconventional superconductivity.
In this work, researchers report the discovery of a pure electron liquid, stabilized on the surface of an electride crystal—an ionic compound where electrons serve as the negatively charged (anionic) component. The crystal’s unique properties enable electrons on its surface to “float,” decoupled from the top layer of atoms, and allow the system to be tuned toward an interesting phase transition.
Previous limitations and a breakthrough
The study of 2D electron phases has been a central focus in this area of inquiry because of its conceptual simplicity and applicability to emergent phenomena such as the Wigner crystal—an exotic solid consisting solely of electrons. A previous approach to realizing a pure 2D electron system involved electrons on the surface of liquid helium (LHe). However, the electron density of such systems is low (1010 cm-2), placing them in the well-studied classical regime.
Here, researchers were able to realize a pure electron system dense enough to place it in the quantum regime, using the surface of an electride material, Gd2C. The material’s interstitial anionic electrons (IAEs) are localized in cavities between [Gd2C]2+ cations. When the surface of this electride is cleaved, the IAEs remain on the surface to maintain charge neutrality with the topmost cationic layer. However, these electrons are no longer localized, but free to move as in a liquid.
2D quantum liquid and induced phase transition
To characterize the floating electrons, the researchers used angle-resolved photoemission spectroscopy (ARPES) at ALS Beamline 4.0.3. The electron density estimated from the data (2×1014 cm-2) was high enough to be in the quantum regime, and the data showed negligible overlap between the floating electron wave function and the orbitals of the top Gd atoms. Other quantities extracted from the data (effective mass, me, and the imaginary part of the self-energy, ImΣ) showed that the electrons were in a liquid state with sizable electron–electron interactions.
ARPES also showed that the deposition of potassium atoms reduces the electron density, driving the system toward an interesting classical-quantum crossover regime thought to harbor rich phenomena. At the lower density, the system undergoes a transition to a state possibly related to a liquid-crystal-like (“hexatic”) phase that has been predicted for Wigner crystals as they melt.
The practical feasibility of this pure electron system—electrons on a rigid crystal surface at relatively high temperature (10 K)—allows its use in various technical approaches, and it is expected to provide an effective platform for gaining insight into intriguing quantum phenomena in the future.
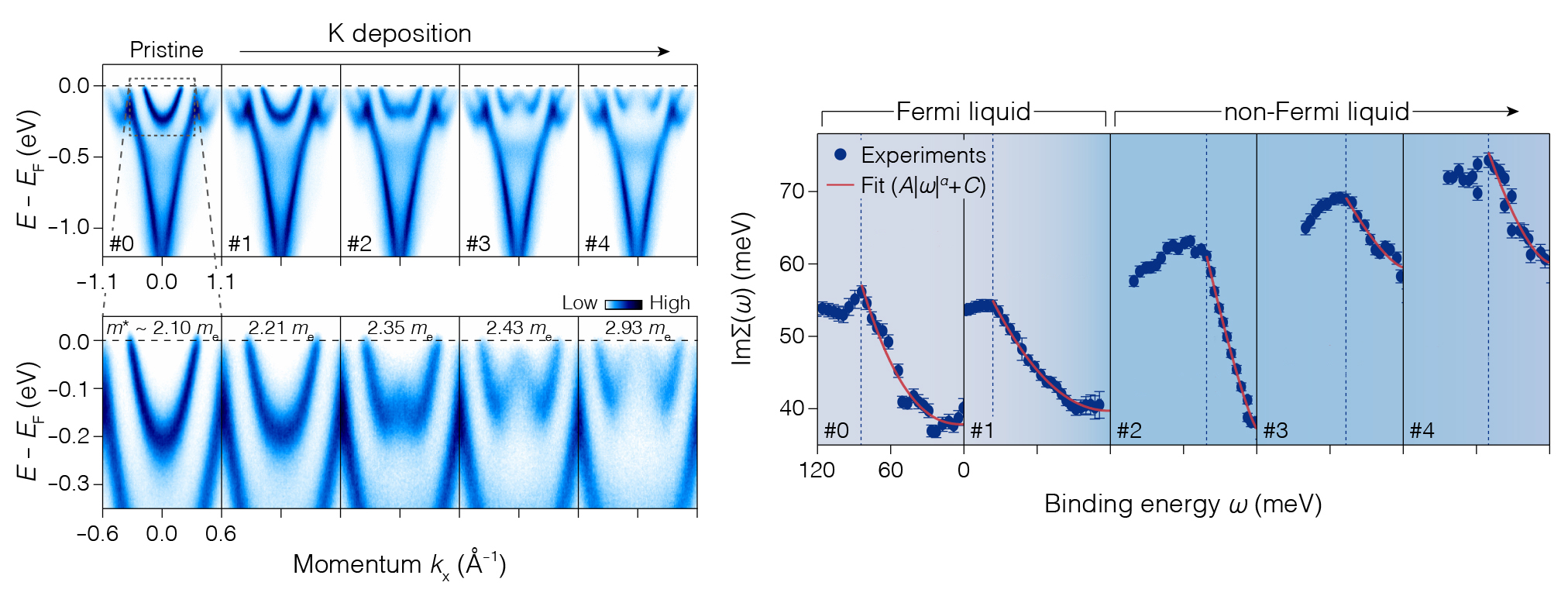
Contact: Yeongkwan Kim
Researchers: S. Kim, C.-y. Lim, J. Hyun, G. Lee, Y. Lee, and Y. Kim (Korea Advanced Institute of Science and Technology); J. Bang and S.Y. Lee (Sungkyunkwan University, Korea); J.D. Denlinger (ALS); S. Huh and C. Kim (Institute for Basic Science and Seoul National University, Korea); S.Y. Song and J. Seo (Daegu Gyeongbuk Institute of Science & Technology, Korea); D. Thapa and S.-G. Kim (Mississippi State University); and Y.H. Lee and S.W. Kim (Sungkyunkwan University and Institute for Basic Science, Korea).
Funding: National Research Foundation of Korea, Samsung Science and Technology Foundation, and Institute for Basic Science (Korea). Operation of the ALS is supported by the U.S. Department of Energy, Office of Science, Basic Energy Sciences program.
Publication: S. Kim, J. Bang, C.-y. Lim, S.Y. Lee, J. Hyun, G. Lee, Y. Lee, J.D. Denlinger, S. Huh, C. Kim, S.Y. Song, J. Seo, D. Thapa, S.-G. Kim, Y.H. Lee, Y. Kim, and S.W. Kim, “Quantum electron liquid and its possible phase transition,” Nat. Mater. 21, 1269 (2022), doi:10.1038/s41563-022-01353-8.
ALS SCIENCE HIGHLIGHT #477