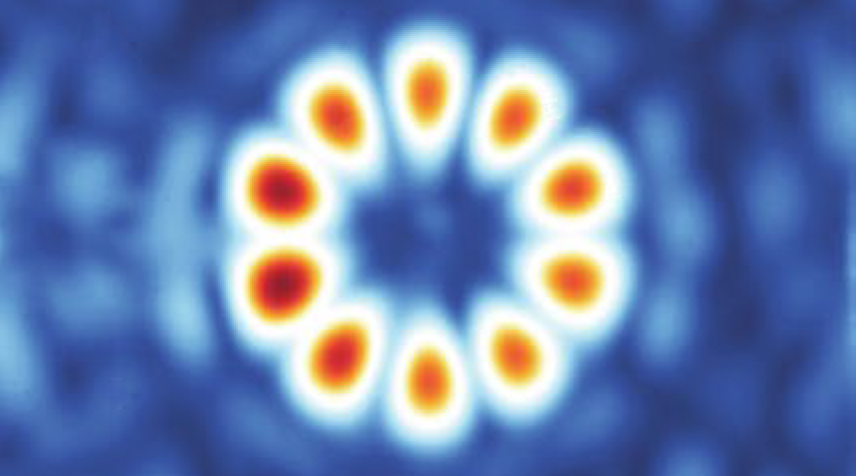
by Lori Tamura
Light waves, when generated a certain way, can exert twisting forces on matter. In the visible-light regime, such beams have been used as “optical tweezers” to trap and manipulate tiny particles (like a tractor beam) or to detect rotational motion in targets. Now, the ability to generate beams with a specific type of rotational character, known as orbital angular momentum (OAM), has been extended to the soft x-ray regime. The work lays the foundation for a new type of soft x-ray contrast mechanism that could provide access to previously hidden material properties.

In a recently published Nature Photonics paper, researchers from the Advanced Light Source (ALS) and the University of Oregon reported on the fabrication and testing of specialized diffraction gratings that, when placed in the coherent light of ALS Beamline 12.0.2, produce OAM soft x-ray beams of exceptionally high quality.
With this work, the researchers have begun to push the envelope of soft x-ray techniques available for probing and manipulating the electronic structure of matter using light. “I’ve been using circularly polarized light for a very long time,” said ALS Staff Scientist and study co-author Sujoy Roy, referring to a commonly used form of light that carries a different kind of angular momentum. For years, researchers have used circularly polarized light to study all kinds of things, from chemical bonds to magnetism. “OAM beams provide a new degree of freedom,” said Roy.
How does OAM light differ from circularly polarized light? In a circularly polarized beam, the electromagnetic field vector rotates around the beam’s central axis. In an OAM beam, it’s the phase of the light wave that rotates, tracing out a helical path as the beam travels through space. This phase structure has some interesting consequences.
Along the beam’s axis, the phase is undefined—like a singularity at the center of a vortex. This causes the beams to appear as a ring, with darkness in the middle. Also, since multiple helices can be intertwined (the double helix of DNA being one example), OAM beams have a “topological charge,” 𝑙, determined by the number of intertwined helices it has.

The topological charge provides a measure of the amount of OAM in a beam: ±𝑙ℏ, where ℏ is the fundamental quantum of angular momentum, and the sign indicates the rotational sense (left or right handed). In this work, the researchers were able to generate a beam carrying an unprecedented 30ℏ of OAM per photon.
They accomplished this by using diffraction gratings with a particular type of defect called a fork dislocation, made at the University of Oregon by study co-authors Spencer Alexander and Ben McMorran. “In my other research with electrons,” said McMorran, “we design and make nanoscale diffraction holograms—patterns of grooves and slits in thin membranes that can imprint a pattern onto a free electron that passes right through it. Here we found that we could use the same structures to manipulate x-rays.”

By perpendicularly crossing two fork gratings, the researchers were also able to create an OAM beam analyzer, which could be used to detect how a beam changes after interaction with a sample. Another type of compound grating allowed the superposition of two types of OAM modes, resulting in diffraction fringes that look like flower petals. “If this beam falls on a sample and elastically scatters, the flower petals will rotate,” said Roy. “But if it scatters inelastically, then the contrast will change.”

The researchers speculate that OAM beams could enable both the manipulation and finer characterization of topologically complex electronic matter, such as magnetic skyrmions or magnetic vortices. “I think if you have a system with some sort of circulation, either a helix or a loop, you can center your vortex beam on that,” said ALS Director and study co-author Steve Kevan. “That’s got to make a difference. We’re always tuning the wavelength to get some different chemical contrast. This is a way to get topological contrast.”
Other ideas include using OAM beams to enhance soft x-ray microscopy, resonant inelastic x-ray scattering (RIXS), or angle-resolved photoelectron spectroscopy (ARPES). There is also the possibility of direct OAM transfer between x-rays and electronic matter.
To work, of course, diffraction gratings require coherent light. With the ALS Upgrade (ALS-U) Project underway, coherent light thousands of times brighter is on the horizon. “With the ALS, you can extract a coherent fraction by throwing out 99% of the beam outside of this one little smooth spot,” said Kevan. “The upgraded ALS will have coherence across the whole beam.”
As more x-ray sources become capable of OAM creation, the researchers’ intent is to establish a model for generating and analyzing soft x-ray OAM beams using diffractive optics. But will this new capability truly enable enhanced contrast? “The jury’s still out,” said Roy. “We will test that. I think for ALS-U science this is a pretty big step,” he said. “It’s really very, very exciting.”
