Scientists have been researching high-temperature (high-Tc) superconductors for decades with the goal of finding materials that express superconducting capabilities at room temperature, which would be a requirement for practical and cost-effective applications. The higher the operating temperature, the more realistic energy-saving applications such as lossless electrical transmission or magnetically levitated trains become. Scientists thought they’d found the key component to high-temperature superconductivity in iron-based materials—that they have a certain type of Fermi surface—but new research at the ALS points to electron correlation as the universal factor.
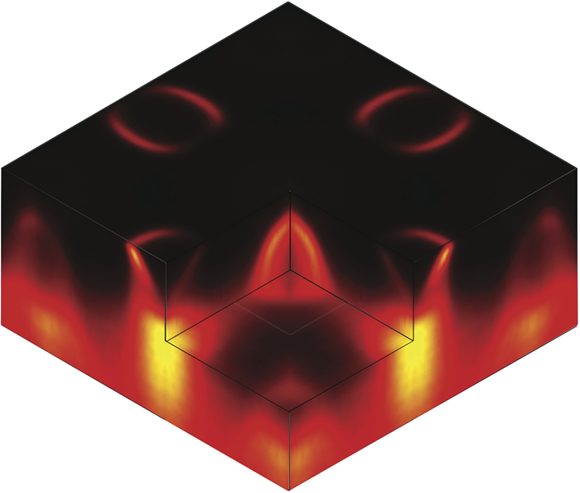
Currently, known high-Tc superconductors are complex materials where the mechanism for superconductivity is still far from clear. The newest materials to gain scientific interest are iron-based superconductors, and the first few years of research have led scientists to believe that these materials all have in common a certain type of Fermi surface, an abstract “map” that defines the allowable energies of electrons in a solid. But recently, researchers from UC Berkeley, Stanford, and Berkeley Lab working at the ALS dispelled that theory. They showed that electron correlation—the strength of interactions of electrons within the material as expressed in the electronic bandwidth—is the essential factor in achieving high Tc in iron-based superconductors. This research sheds light on a new and important quality of these promising materials and moves them one step closer to commercial application.
Most iron-based superconductors combine a conducting layer of iron with a pnictogen (chemical elements in Group 15 of the periodic table), typically arsenic or phosphorous. These types of iron-based superconductors, discovered just eight years ago, provided a second class of high-temperature superconductors (the first being copper-based cuprates), which has been helpful for determining the minimal ingredients necessary for superconductivity.
It was in this vein of thinking that researchers set out to look at an even newer subclass of iron-based materials called iron chalcogenides, which combine iron and a chalcogen (sulfur, selenium, or tellurium). Scientists studying iron-based superconductors previously had concluded that a necessary ingredient for superconductivity was a certain type of Fermi surface, essentially a map of electronic states in a material that governs its properties. Studies had noted that iron-based superconductors had a set of hole Fermi surfaces and a set of electron Fermi surfaces that were separated such that electrons could scatter from one to another. The widely accepted theory was that it was magnetic fluctuations between these two sets of Fermi surfaces that mediated superconductivity.
However, recent research at ALS Beamline 10.0.1 using angle-resolved photoemission spectroscopy (ARPES) has shown that the specific Fermi surfaces previously observed do not have to be present in order for iron-based materials to perform as high-Tc superconductors. The iron chalcogenide materials that ALS researchers studied only had electron Fermi surfaces (they were missing the hole Fermi surface that most iron-based superconductors have) and yet were still observed to superconduct at a relatively high temperature.
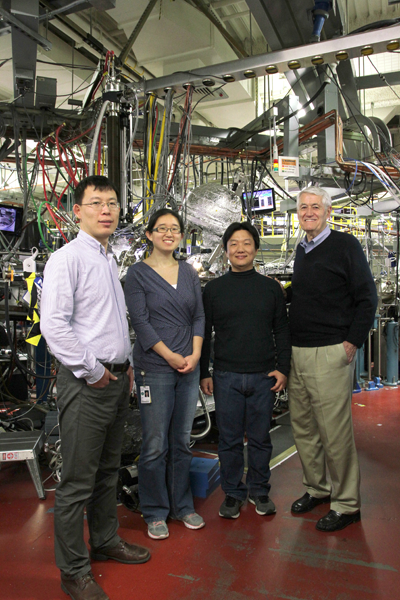
More importantly, the researchers found that it was not the shape of the Fermi surfaces that dictated the superconducting properties of the materials. The three different kinds of samples they studied ranged from a non-superconductor to a superconductor that had a transition temperature (Tc) of 32 K, yet they all had the same Fermi surfaces. Instead, the characteristic that distinguished superconducting characteristics in the iron chalcogenide samples was their electronic bandwidth, which is a measure of the strength of electron interactions in these compounds. The high flux available at Beamline 10.0.1 allowed researchers to chart the slope of the electronic bands in their various iron chalcogenide samples. The band slopes, which indicate the velocity at which electrons travel in the materials, were much lower in the samples that had the highest levels of superconductivity. The lower band slope reveals that the electrons travel more slowly due to the strong interaction with other electrons in the materials. From the non-superconducting sample to the 32 K superconductor, the band slope changed by a factor of two, revealing that the strength of electron interaction is an important and necessary factor for the occurrence of superconductivity in the iron-based superconductors.
It has been known that the copper-based high-Tc materials are very strongly correlated. However, the role of electron correlation has not been given as much attention for the iron-based superconductors due to the previous attention on the common Fermi surface topology. This work shows that looking at electron correlations could be one of the most significant methods of understanding and discovering new and existing high-Tc materials.
Contact: Ming Yi
Research conducted by: M. Yi, Meng Wang (UC Berkeley); A. F. Kemper (Computational Research Division, Lawrence Berkeley National Laboratory); S.-K. Mo, Z. Hussain (Advanced Light Source); E. Bourret-Courchesne (Materials Science Division, Lawrence Berkeley National Laboratory); A. Lanzara (UC Berkeley, Materials Science Division, Lawrence Berkeley National Laboratory); M. Hashimoto, D. H. Lu (Stanford Synchrotron Radiation Lightsource); Z.-X. Shen (Stanford University); and R. J. Birgeneau (UC Berkeley, Lawrence Berkeley National Laboratory).
Research funding: U.S. Department of Energy (DOE), Office of Basic Energy Sciences (BES). Operation of the ALS is supported by DOE BES.
Publication about this research: M. Yi, Meng Wang, A. F. Kemper, S.-K. Mo, Z. Hussain, E. Bourret-Courchesne, A. Lanzara, M. Hashimoto, D. H. Lu, Z.-X. Shen, and R. J. Birgeneau, “Bandwidth and Electron Correlation-Tuned Superconductivity in Rb0.8Fe2(Se1 -zSz)2,” Phys. Rev. Lett. 115, 256403 (2015).
ALS SCIENCE HIGHLIGHT #329