For materials scientists and physicists, weakly interacting two-dimensional layers are ideal building blocks. Stacking such materials in clever ways can produce heterostructures that exhibit novel, potentially beneficial properties not found in any of the constituent materials alone. To explore this phenomenon, researchers performed angle-resolved photoemission spectroscopy (ARPES) studies at the ALS on epitaxial graphene deposited on a substrate of hexagonal boron nitride (h-BN). The results directly reveal for the first time that interaction between the graphene and h-BN alters electronic states in the heterostructure, generating new spectral features (i.e., second-generation Dirac cones) and inducing a bandgap in the graphene, greatly improving its suitability for advanced, ultralow-power device applications.
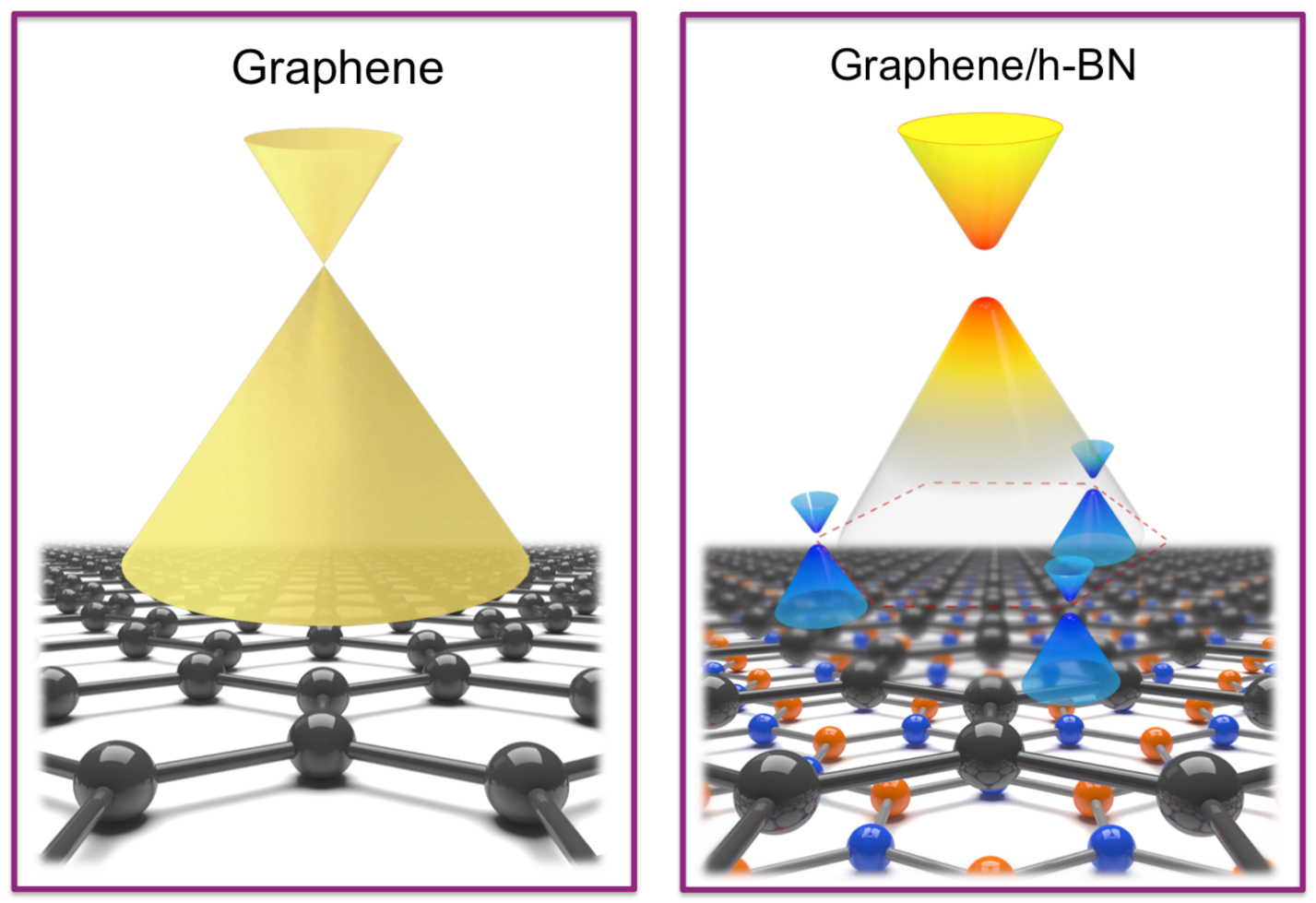
Graphene and h-BN both have honeycomb-like hexagonal lattice structures. However, the h-BN honeycomb is slightly larger, by 1.8%. Therefore, a periodic moiré pattern forms when these two materials are layered together. With a relatively large periodicity (about 56 times the normal graphene crystal periodicity), this moiré pattern acts as a superlattice, effectively applying a more slowly varying periodic potential to the system. In addition, because the boron and nitrogen atoms in h-BN differ from the carbon in graphene, inversion symmetry is broken in this heterostructure.
The effects of the superlattice potential on the electronic properties of graphene/h-BN have been predicted to result in various novel quantum phenomena, including the emergence of a new set of “second-generation” Dirac cones (which are crucial to the realization of Hofstadter butterfly states under an applied magnetic field), and the opening of bandgaps at Dirac points (necessary if graphene is to be used in practical devices). However, the locations and shapes of the second-generation Dirac cones and the effect of inversion symmetry breaking on the opening of gaps have been highly debated.
Previous studies provided evidence for the existence of second-generation Dirac cones, but it was mainly indirect evidence derived from scanning tunneling microscopy and transport measurements, which provide energy-resolved information only, leaving the momentum component ambiguous. The results also led to controversial conclusions about the existence of bandgaps.
Using ARPES at ALS (formerly Beamline 12.0.1, now Beamline 10.0.1), researchers were able to reveal directly for the first time the electronic spectrum of second-generation Dirac cones and resolve gaps induced by the breaking of inversion symmetry. The momentum separation between the first- and second-generation Dirac cones was on the order of 0.05 Å-1, which is only around 2% of the graphene Brillouin zone. Thus, to resolve the detailed band structure, they needed very high quality ARPES data from a high-quality sample with an extremely clean surface. They successfully developed an effective process for obtaining clean samples after a year of trial and error. The flexibility of the small preparation chamber at the beamline proved valuable in easing the learning curve.
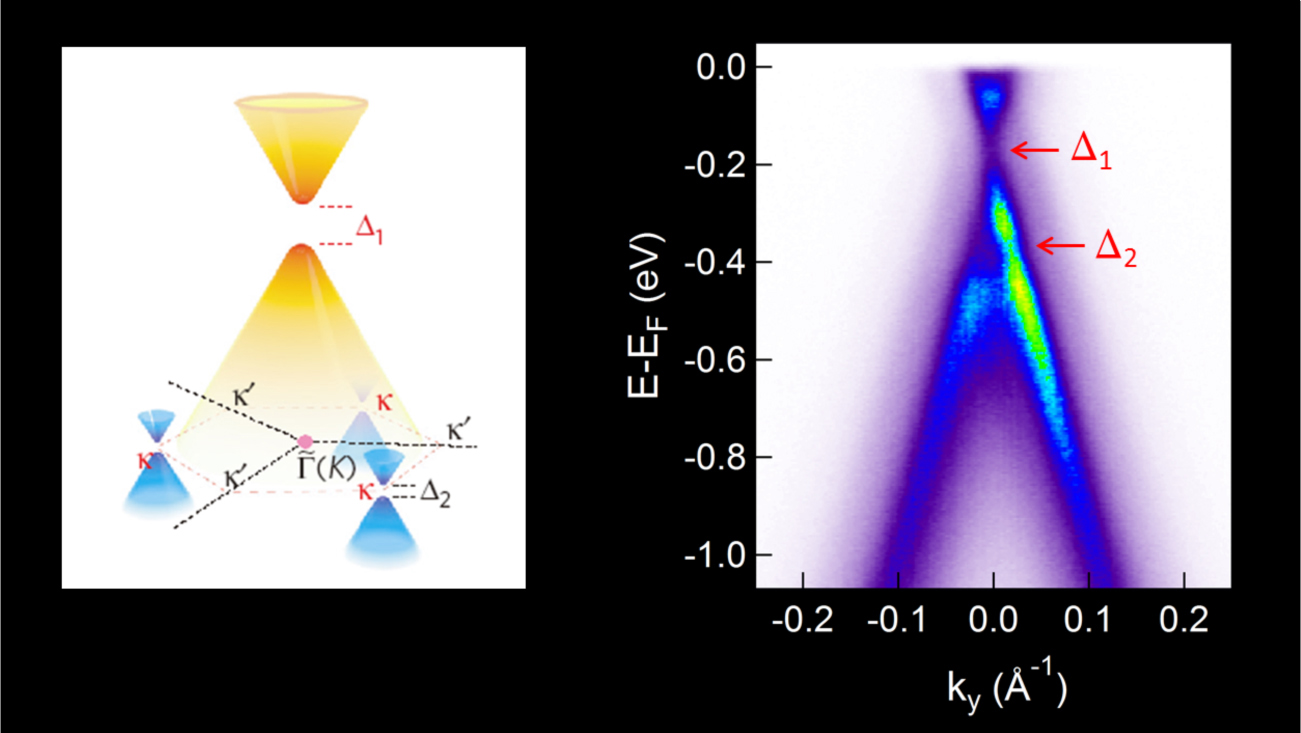
The results showed that the second-generation Dirac cones were located at the superlattice Brillouin zone corners and at one of its two valleys. Due to the inversion symmetry breaking, gaps of about 160 and 100 meV were observed at the first- and second-generation Dirac points, respectively. The gap sizes of both were much larger than predicted theoretically, indicating that the interaction between the graphene and h-BN was stronger than previously expected.
The work conclusively demonstrates that layering graphene and h-BN effectively modifies the electronic properties of the graphene, making it more suitable for device applications than graphene alone. More generally, the work highlights the important role of a strong inversion-symmetry-breaking perturbation potential in the physics of graphene/h-BN and fills critical knowledge gaps in the band-structure engineering of Dirac fermions by a superlattice potential. More functionality from stacking such two-dimensional building blocks awaits discovery.
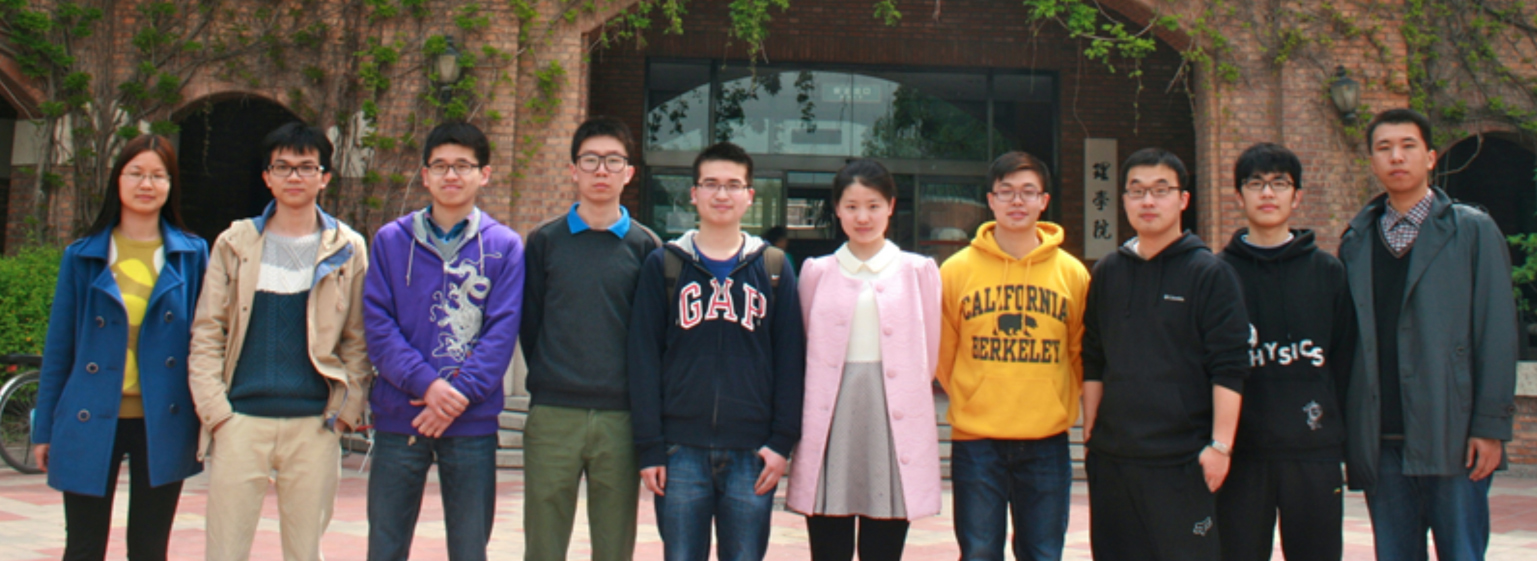
Contact: Shuyun Zhou
Research conducted by: E. Wang, S. Ding, W. Yao, M. Yan, G. Wan, and K. Deng (Tsinghua University, China); X. Lu and S. Wang (Chinese Academy of Sciences); G. Chen, L. Ma, and Y. Zhang (Fudan University, China); J. Jung (University of Seoul); A.V. Fedorov (ALS); G. Zhang (Chinese Academy of Sciences and Collaborative Innovation Center of Quantum Matter, China); and S. Zhou (Tsinghua University and Collaborative Innovation Center of Quantum Matter, China).
Research funding: National Natural Science Foundation of China, Ministry of Science and Technology of China, Tsinghua University Initiative Scientific Research Program, and Advanced Light Source Doctoral Fellowship Program. Operation of the ALS is supported by the U.S. Department of Energy, Office of Basic Energy Sciences.
Publication about this research: E. Wang, X. Lu, S. Ding, W. Yao, M. Yan, G. Wan, K. Deng, S. Wang, G. Chen, L. Ma, J. Jung, A.V. Fedorov, Y. Zhang, G. Zhang, and S. Zhou, “Gaps induced by inversion symmetry breaking and second-generation Dirac cones in graphene/hexagonal boron nitride,” Nat. Phys. 12, 1111 (2016). doi:10.1038/nphys3856
SCIENCE HIGHLIGHT #344