Researchers have discovered that “Fermi arcs,” which are much-debated features found in the electronic structure of high-temperature superconducting (HTSC) cuprates, can also be found in an iridate (iridium oxide) compound. At the ALS, the researchers observed the electronic structure of strontium iridate as it evolved through different doping levels and temperatures by using angle-resolved photoemission spectroscopy (ARPES) with in situ electron doping of the sample surface. The existence of a “strange” metallic phase with Fermi arcs and a pseudogap suggests the possible emergence of high-temperature superconductivity in this non-cuprate compound.
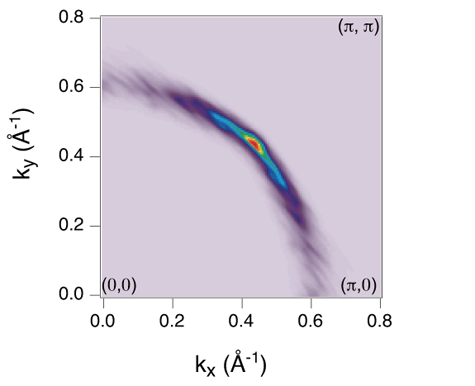
In conventional superconductors, the superconducting gap—an indicator of superconductivity—vanishes above the superconducting transition temperature (Tc). In HTSC cuprates, however, the gap can be seen above Tc and has been dubbed the “pseudogap,” as its origin is, so far, unknown; the explanation of this mystery is expected to contain crucial information about the HTSC mechanism. “Fermi arcs” are another remarkable cuprate feature: these are fragments of the Fermi surface that retract and become isolated from each other as temperature and doping decrease. Because Fermi arcs cannot be regarded as a normal (continuous) Fermi surface, the Fermi-arc phase is regarded as a “strange” metallic phase that cannot be explained by a general understanding of electron behavior in a normal metal.
Although explanations of the pseudogap and Fermi arcs remain open to debate, scientists in the HTSC field commonly believe that certain unique cuprate features are essential to superconductivity: spin-1/2 moment on a quasi-two-dimensional square lattice, Heisenberg antiferromagnetic coupling, and no orbital degeneracy. A minimal model based on these assumptions can reproduce much of the phenomenology of HTSC cuprates. Although it would be informative to study these key features in a non-cuprate sample, so far no ideal material has been found.
The 5d transition-metal oxide, strontium iridate (Sr2IrO4), is the most promising candidate, exhibiting all three of the above features. As a result, its effective low-energy physics can be described by the same minimal model as for cuprates, suggesting that it will also be a possible unconventional HTSC upon charge-carrier doping. However, very little is known about what occurs in the electronic phases of strontium iridate when additional carriers are provided.
To address this issue, the researchers performed at ALS Beamline 4.0.3 a complete ARPES experiment, directly mapping out the electronic structure using ultraviolet light. They employed an in situ doping technique that deposited potassium atoms on the sample surface, adding electrons to the system. By controlling the surface coverage (i.e., the doping level), the researchers succeeded in revealing the evolution of the electronic structure from a Mott insulator to a normal metallic phase. At intermediate coverage, they observed a Fermi arc and related pseudogap that resembled that of an HTSC cuprate. Further temperature- and coverage-dependent studies showed that the parallel between strontium iridate and cuprates persists in the metallic phase and that the breakup of a Fermi surface into segments is a general phenomenon of a system containing certain generic cuprate characteristics.
The nature of the phase that manifests Fermi arcs in electron-doped strontium iridate is unclear at this point. A growing body of evidence in cuprates suggests that Fermi arcs and pseudogaps are associated with distinct phases in HTSCs, such as density waves, stripes, and checkerboard orders. If so, it would prompt investigation of such phases in carrier-doped strontium iridate. If, on the other hand, Fermi arcs and pseudogaps are precursory signatures of high-temperature superconductivity, strontium iridate may become superconducting. In any case, the results suggest that strontium iridate is a useful model system for reproducing the physics expected in HTSC cuprates. The researchers expect that further study of this material will shed new light on the long-sought connection between the pseudogap and high-temperature superconductivity.
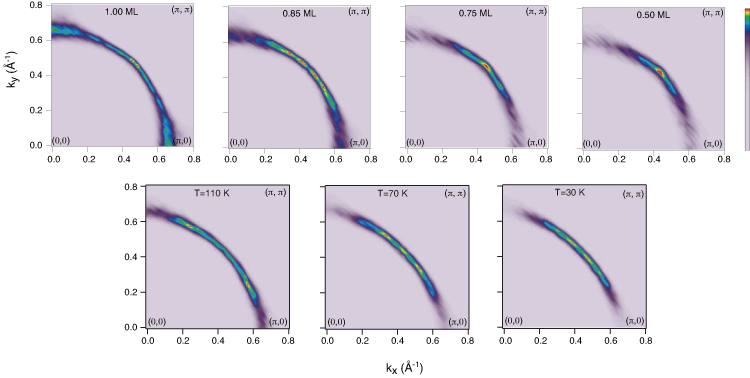
Contact: Yeongkwan Kim
Research conducted by: Y.K. Kim, O. Krupin, J.D. Denlinger, A. Bostwick, and E. Rotenberg (ALS); Q. Zhao and J.F. Mitchell (Argonne National Laboratory); J.W. Allen (Univ. of Michigan); and B.J Kim (Argonne National Laboratory, Univ. of Michigan, and Max Planck Institute for Solid State Research).
Research funding: National Science Foundation, National Research Foundation of Korea, and U.S. Department of Energy (DOE), Office of Basic Energy Sciences (BES). Operation of the ALS is supported by DOE BES.
Publication about this research: Y.K. Kim, O. Krupin, J.D. Denlinger, A. Bostwick, E. Rotenberg, Q. Zhao, J.F. Mitchell, J.W. Allen, and B.J Kim, “Fermi arcs in a doped pseudospin-1/2 Heisenberg antiferromagnet,” Science 345, 187 (2014).
ALS SCIENCE HIGHLIGHT #301