Materials that can both conduct and insulate against the flow of electricity are of great interest for use as components in advanced electronic devices as well as for fundamental condensed-matter studies. Nearly 50 years ago, the transition-metal oxide V2O3 was found to qualify as such a material, being a conductor (i.e., metallic) above 160 K and an insulator below. Despite progress in methods to model these observations theoretically, the lack of momentum-resolved photoemission data to verify the theories meant that the origins of this phenomenon ultimately remained a mystery. Now, researchers report on angle-resolved photoemission spectroscopy (ARPES) measurements performed on samples of V2O3. The results overturn a decade-old theory about metal–insulator transitions (MITs) in this material and provide a spectroscopic benchmark test for future models.
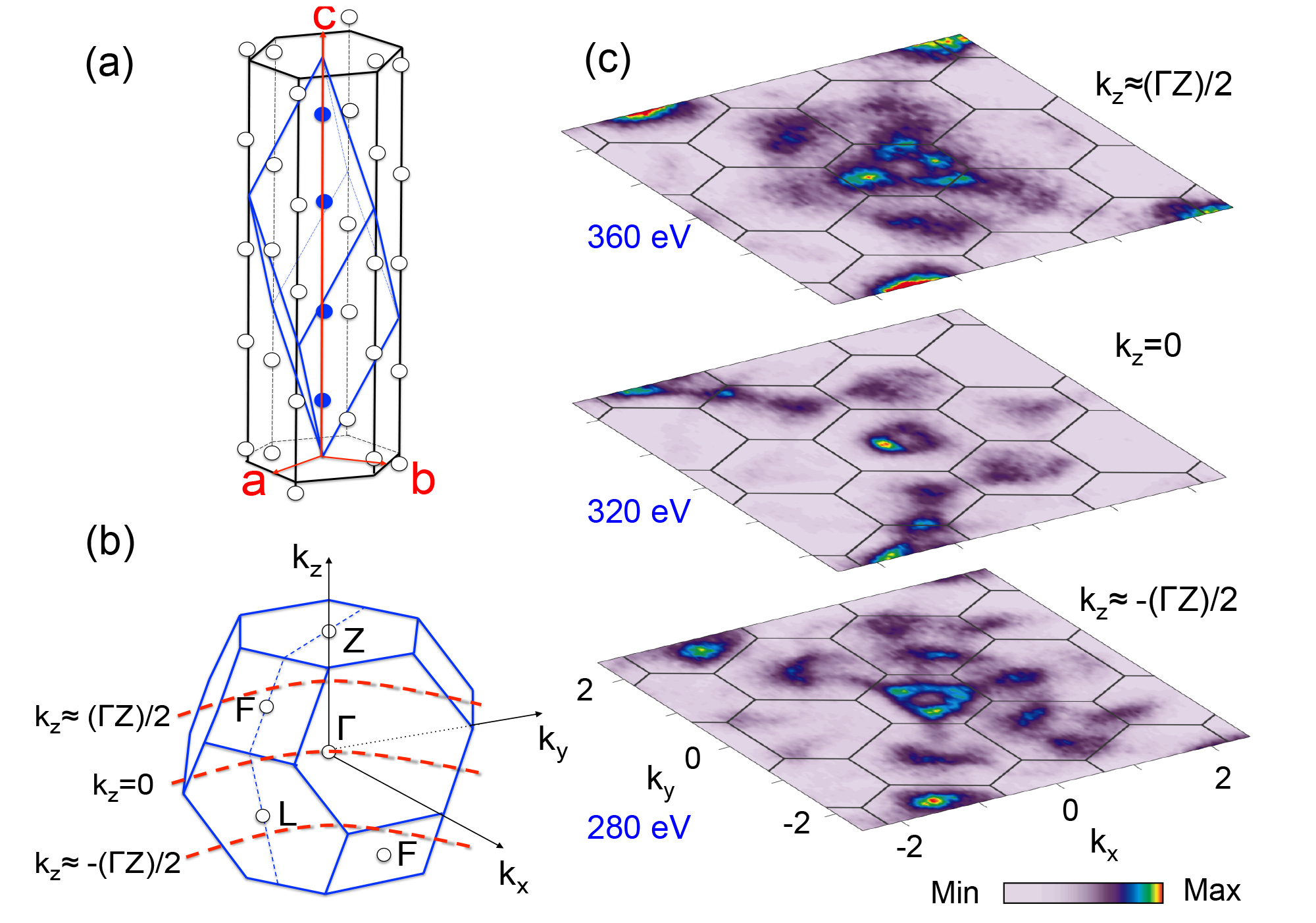
Initially, V2O3 was thought to be a real-world example of an ideal Mott MIT, in which the insulator state arises as a result of Coulomb repulsion between electrons. However, this was quickly challenged on the grounds that it didn’t take into account the material’s multi-orbital electronic structure: each trivalent vanadium ion has two 3d electrons distributed between two overlapping d-orbitals and
.
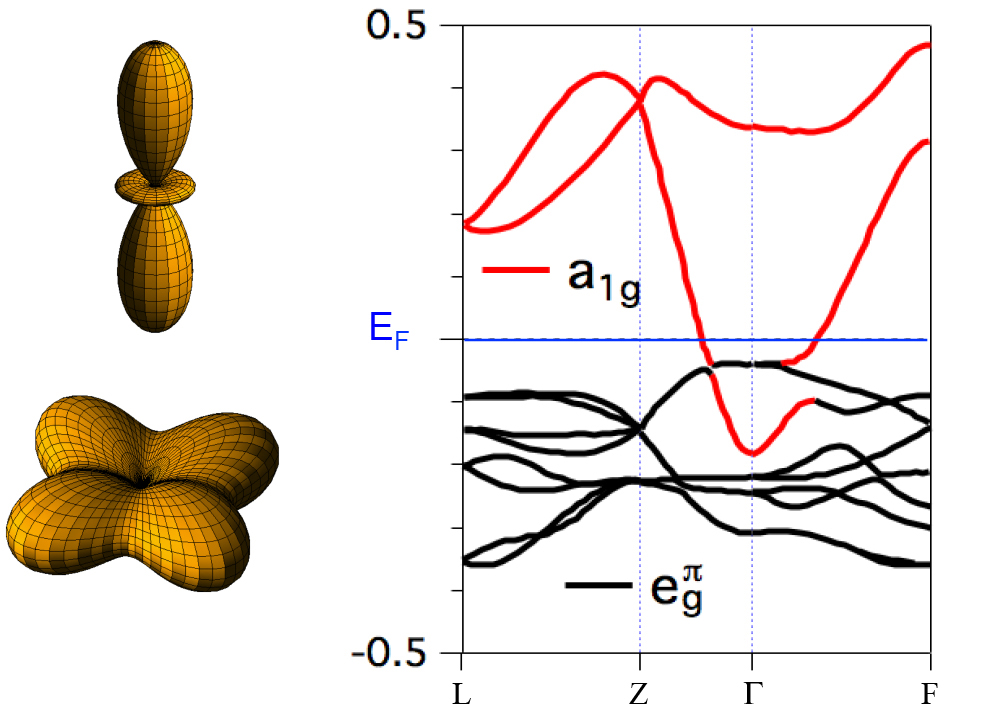


In the late 1990s, the integration of density functional theory (DFT) with dynamical mean-field theory (DMFT) gave real hope of solving the mystery within a realistic multiorbital calculation. Bulk-sensitive angle-integrated photoemission provided a basic confirmation of the DMFT theory, but was not able to discriminate between the details of different DFT+DMFT theories.
A series of DFT+DMFT studies in the early 2000s coalesced in 2007 around a particular model in which Coulomb repulsion forces drive the splitting of the and
states in the metallic phase to the brink of an insulating gap near the MIT. Small structural perturbations from temperature changes and doping would then be needed to push the system over the transition. The model’s predictions included a Fermi surface featuring a single
electron pocket, as well as completely filled
bands. Over time, however, technical advances in the DFT+DMFT calculations began to show discrepancies with the 2007 model, setting the stage for the next logical development: measurement of the momentum-resolved band structure of V2O3.
Scientists at the ALS performed ARPES experiments on single-crystal V2O3 samples using the 2009 configuration of Beamline 7.0.1 (the ARPES program has since moved to Beamline 4.0.3). The wide-angle detection capability of the beamline’s electron spectrometers was important for characterizing the weak-contrast, angle-dependent, band-structure changes in the rather broad features inherent in the V2O3 spectra. The measurements were taken at a temperature of 200 K, fully in the metallic phase.
The measurement of a triangular Fermi surface with a hole-like band dispersion—as opposed to an electron pocket—was the crucial observation directly contradicting the 2007 theory. While the key Fermi surface and band dispersion data were acquired at a photon energy of 180 eV, the wide photon-energy range of Beamline 7.0.1 (80–900 eV) also allowed the verification of the triangular Fermi surface feature at more bulk-sensitive ranges, thus helping to prove the bulk origin of features that were measured at lower, more surface-sensitive, energies.
To support their findings and gain greater insight, the researchers performed band calculations using DFT+U methods (U is a parameter accounting for the Coulomb repulsion between electrons). A match was found for the specific half-filling of the band observed in the data. The calculations also gave rise to a Fermi-surface contour with an open-tipped triangular feature very similar in size and shape to that obtained via the ARPES experiments. In the future, analysis using the more-sophisticated DMFT will be needed to fully explain the details of the experimental data and to provide a new explanatory mechanism for the metal–insulator transition in V2O3.
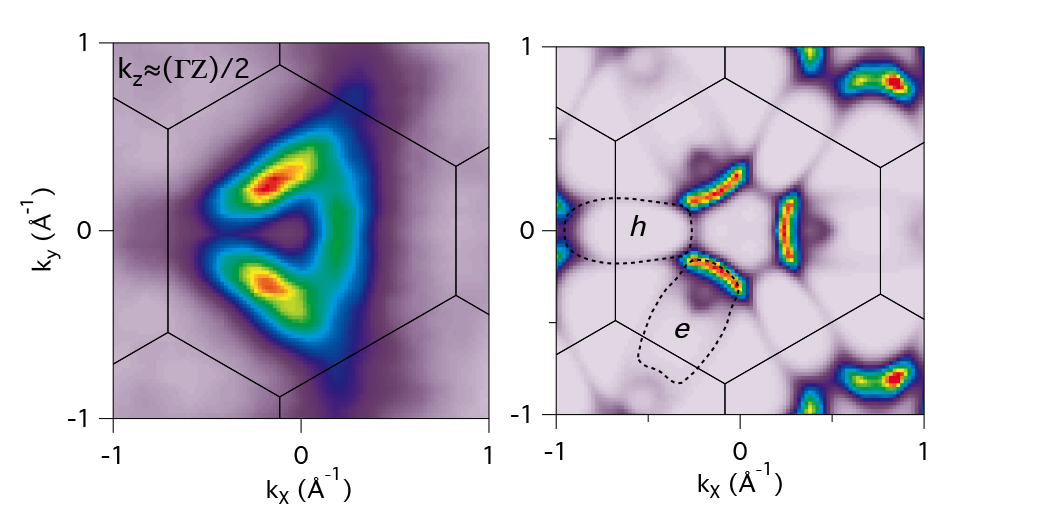
Contact: Jonathan Denlinger
Research conducted by: I. Lo Vecchio (Berkeley Lab), J.D. Denlinger and O. Krupin (ALS), B.J. Kim (Max Planck Institute for Solid State Research, Germany), P.A. Metcalf (Purdue University), S. Lupi (Sapienza University of Rome), J.W. Allen (University of Michigan), and A. Lanzara (Berkeley Lab and UC Berkeley).
Research funding: National Science Foundation. Operation of the ALS is suppoerted by the U.S. Department of Energy, Office of Basic Energy Sciences.
Publication about this research: I. Lo Vecchio, J.D. Denlinger, O. Krupin, B.J. Kim, P.A. Metcalf, S. Lupi, J.W. Allen, and A. Lanzara, “Fermi Surface of Metallic V2O3 from Angle-Resolved Photoemission: Mid-level Filling of Bands,” Phys. Rev. Lett. 117, 166401 (2016). doi: 10.1103/PhysRevLett.117.166401
ALS SCIENCE HIGHLIGHT #348