Magnetic memory technology involves the reading and writing of magnetic binary states. Although the difficulties involved in reading small-sized magnetic bits have been successfully overcome, the writing aspect (i.e., the controlled flipping of magnetic binary states) still poses a challenge. At the ALS, photoemission electron microscopy (PEEM) experiments have demonstrated that the circulation of a magnetic vortex—a promising form of magnetic binary state—can be made to switch directions through the application of an electric field. The discovery opens the door to digital devices with more streamlined system designs, improved performance, and greater energy efficiency.
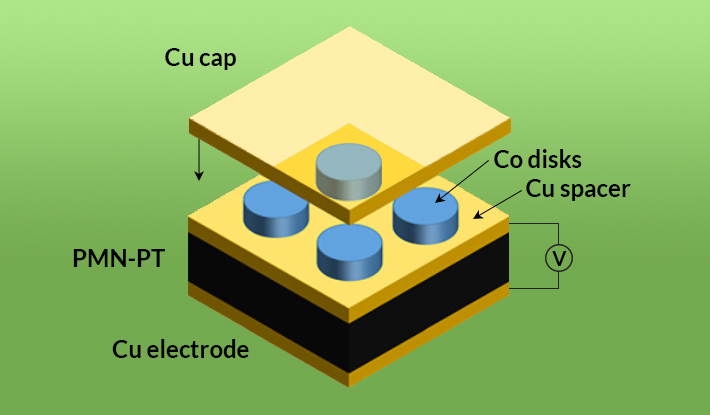
If a thin magnetic film is constrained in size and shape to a disk about a micrometer in diameter, its magnetization vectors will form a spiral, or “vortex,” with either a clockwise or counterclockwise circulation. The binary nature of these magnetic vortex states makes them a possible mechanism for a type of magnetic memory that would be fast, compact, and nonvolatile. It would be able to handle the full range of tasks performed in modern digital devices, potentially replacing several types of memory technologies with one “universal” memory.
Many previous studies have demonstrated the controlled switching of vortex circulation by various magnetic means, including the introduction of an asymmetry in the disk shape, an asymmetrically applied magnetic field, nanosecond magnetic field pulses, and exchange bias. On the other hand, there have been few reports, either theoretical or experimental, of the controlled switching of vortex states by an electrical field, a process that dissipates less energy as heat and would therefore be more energy efficient.
A promising electric-field-based approach involves inducing strain in the crystal structure of a ferromagnetic film through contact with a piezoelectric substrate (i.e., one that changes shape in an electric field). The strain transmitted from the substrate would stretch or contract the film’s crystal lattice, affecting its easy-magnetization axis (the energetically favorable direction for spontaneous magnetization), leading in turn to the flipping of any vortex states present. To explore this phenomenon, the researchers fabricated thin, ferromagnetic cobalt (Co) disks, 1.5 µm in diameter, on a piezoelectric substrate of lead magnesium niobate–lead titanate (PMN-PT).
At the ALS, the researchers conducted magnetic spectroscopy experiments at Beamline 6.3.1, where a 2.0 T magnet made it possible to measure magnetic hysteresis loops and to saturate the disks’ vortex cores in one direction. PEEM images of the samples were then obtained at Beamline 11.0.1.1, where circularly polarized x-rays at the Co L3,2 edges were used to distinguish the different absorption intensities for magnetization parallel and antiparallel to the x-ray beam (a contrast mechanism known as x-ray magnetic circular dichroism, or XMCD). Because PEEM measurements require the sample to be at high voltage, the researchers included a copper (Cu) spacer layer between the Co disks and the PMN-PT substrate, with a thinner Cu cap on top to allow electrons to escape for the PEEM measurements. A series of images were taken with an applied voltage increasing from 0 to 0.8 MV/m in steps of 0.05 MV/m.
The researchers observed that the vortex circulation switched at electrical fields between 0.2 and 0.5 MV/m, corresponding to the PMN-PT’s ferroelectrical coercivity (the electrical field necessary to change the direction of the electric polarization, equivalent to the magnetic field necessary to change the magnetization direction of magnetic materials). The results clearly demonstrate that the electrical switching of the magnetic vortex circulation in the Co disks is the result of strain-induced changes transmitted from the substrate. Furthermore, the magnitude of the transferred strain can be tuned by inserting Cu spacers of various thicknesses between the Co layer and the substrate. In future experiments, the researchers plan to apply electrical pulses with controlled amplitude, duration, and shape to reveal the relationship between vortex circulation switching and time-varying strain.
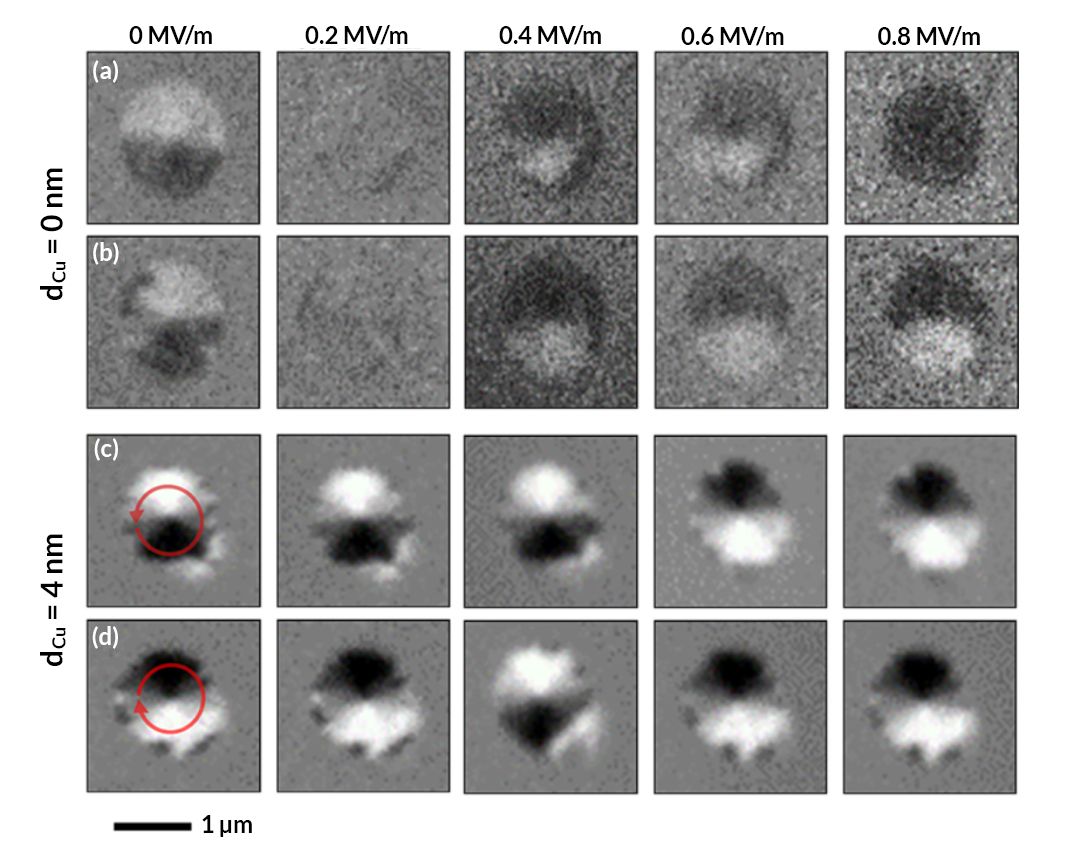
Contact: Z.Q. Qiu
Research conducted by: Q. Li, A. Tan, M. Yang, and Z.Q. Qiu (Univ. of California, Berkeley); A. Scholl, A.T. Young, A.T. N’Diaye, and E. Arenholz (ALS); C. Hwang (Korea Research Institute of Standards and Science); and J. Li (Peking University).
Research funding: National Key Research and Development Program of China, National Science Foundation, and National Research Foundation of Korea. Operation of the ALS is supported by the U.S. Department of Energy, Office of Science, Basic Energy Sciences Program.
Publication about this research: Q. Li, A. Tan, A. Scholl, A.T. Young, M. Yang, C. Hwang, A.T. N’Diaye, E. Arenholz, J. Li, and Z.Q. Qiu, “Electrical switching of the magnetic vortex circulation in artificial multiferroic structure of Co/Cu/PMN-PT(011),” Appl. Phys. Lett. 110, 262405 (2017). doi:10.1063/1.4990987
ALS SCIENCE HIGHLIGHT #360