SCIENTIFIC ACHIEVEMENT
An innovative infrared-light probe with nanoscale spatial resolution has been expanded to cover previously inaccessible far-infrared wavelengths.
SIGNIFICANCE AND IMPACT
The ability to investigate heterogeneous materials at nanometer scales and far-infrared energies will benefit a wide range of fields, from condensed matter physics to biology.
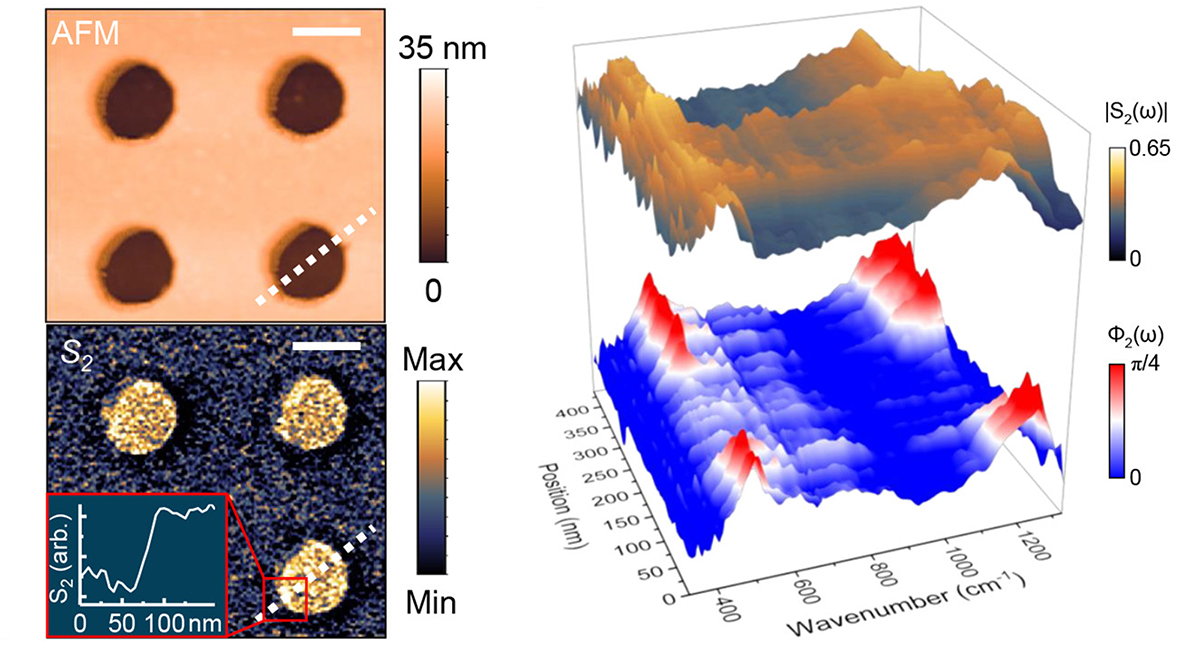
Far-infrared blind spot
Scientific studies require tools that match the natural length and energy scales of the phenomena under investigation. For many questions in biology, quantum materials, and electronics, this means nanometer spatial resolution combined with far-infrared energies. For example, scientists might want to study collective electron oscillations in quantum materials for optoelectronic circuits, or the characteristic vibration modes of protein molecules in biological systems.
A recently developed infrared technique—synchrotron infrared nanospectroscopy (SINS)—combines broadband synchrotron light with atomic-force microscopes to enable infrared imaging and spectroscopy at the nanoscale. However, the technique could only be used in a narrow range of the electromagnetic spectrum that excluded far-infrared wavelengths, due to a scarcity of suitable light sources and detectors for that range. In this work, researchers extended SINS to far-infrared wavelengths, opening up a whole new experimental regime.
The expansion of SINS
SINS was successfully demonstrated at the ALS in 2014 and has subsequently been developed into user endstations at Beamlines 2.4 and 5.4. In this technique, infrared light is focused onto the sharp metallic tip of an atomic force microscope (AFM). The backscattered light from the tip and sample surface is collected and sent to a detector. The spatial resolution of the technique is determined to first approximation by the radius of the tip apex, typically 10–20 nm, much smaller than the wavelength of the light (i.e., it overcomes the diffraction limit by >1000x).
The usable wavelength range is determined primarily by the optical elements and detector, typically mercury-cadmium-telluride (MCT) types, which cut off at wavelengths below 20 µm, not quite reaching the longer wavelengths of the far infrared. Conventional far-infrared detectors are available, but are too slow for use with typical AFM-tip oscillation frequencies. To overcome these issues, the researchers developed a fast, highly sensitive, copper-doped germanium (Ge:Cu) detector that extends the SINS usable wavelength range to 31 µm.

Phonons, plasmons, and beyond
To demonstrate the applicability and generalizability of the technique, the researchers measured representative functional materials from different classes, including dielectrics and polar oxides, organic and molecular systems, and ultrathin 2D van der Waals materials. They probed numerous behaviors and excitations, including lattice vibrations (phonons), free-electron oscillations (plasmons), and molecular vibrations. Distinct spectroscopic signatures for a variety of materials were resolved with high sensitivity to parameters like thickness, crystal orientation, and applied voltage.
In particular, the researchers demonstrated the ability to control and tune the far-infrared, nanoscale plasmonic properties of a graphene device, modulated by an applied electrostatic gate voltage. Graphene attracts immense scientific attention because of its inherent tunability and unique optical and infrared properties, which could potentially be exploited for applications in telecommunication, medicine, and homeland security. The results open up the possibility of new investigations into graphene as well as other novel 2D materials at far-infrared wavelengths.
The researchers are continuing to push the technique to cover the entire infrared range, extending all the way to terahertz frequencies. Within this spectral range, there are a host of novel materials with intriguing properties that would otherwise be inaccessible.

Contact: Omar Khatib
Researchers: O. Khatib (University of Colorado Boulder and ALS), H.A. Bechtel and M.C. Martin (ALS), M.B. Raschke (University of Colorado Boulder), and G.L. Carr (Brookhaven National Laboratory).
Funding: National Science Foundation; U.S. Department of Energy, Office of Science, Basic Energy Sciences Program (DOE BES). Operation of the ALS is supported by DOE BES.
Publication: O. Khatib, H.A. Bechtel, M.C. Martin, M.B. Raschke, and G.L. Carr, “Far Infrared Synchrotron Near-Field Nanoimaging and Nanospectroscopy,” ACS Photonics 5, 2773 (2018), 10.1021/acsphotonics.8b00565.
ALS SCIENCE HIGHLIGHT #385