Recent research at ALS Beamline 5.3.1, detailed in this month’s Science Highlight, revealed that an important photosynthetic mechanism called “nonphotochemical quenching” is triggered by the translocation of the carotenoid pigment within a critical light-sensitive protein called the Orange Carotenoid Protein (OCP). The x-ray footprinting (XFP) technique developed at 5.3.1 allowed researchers to confirm that this translocation actually occurs when OCP is in its natural solution environment and was not due to structural changes that the protein undergoes during crystal formation.
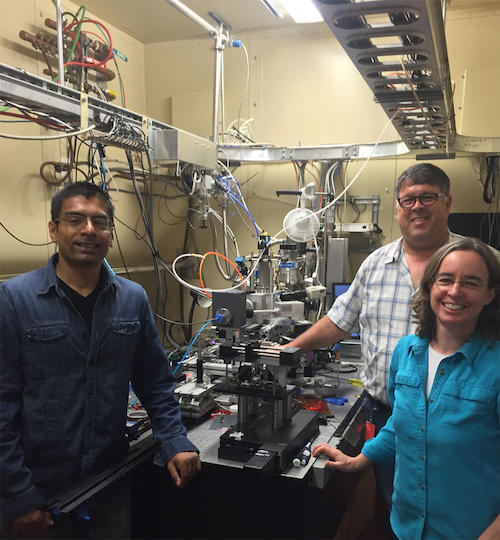
XFP, a powerful technique for the study of macromolecular structures and dynamics of proteins and nucleic acids in solution, is relatively new to the ALS, though it was first developed more than 10 years ago by scientist Mark Chance at the NSLS. Two years ago, Head of the Berkeley Center for Structural Biology (BCSB) Corie Ralston obtained LDRD funding to bring the technique to the ALS, partly based on the fact that the only other XFP capability available in the U.S. was at the NSLS, and soon to be unavailable due to NSLS closure. The ALS is currently supporting NSLS users while they recommission their own XFP beamline.
“The user base is also big enough that we need more than one beamline with XFP capability,” says Ralston. “Even once NSLS is back up in 2016, we plan to continue to offer the technique.”
Ralston describes XFP as being “highly complementary to crystallography,” though one of its main advantages happens to be that it doesn’t require a crystal. Using XFP, researchers can look at proteins in solution and see where the protein is solvent-accessible. “So if you want to look at a protein-protein interaction, for example, where the proteins meet they exclude solvent and you get a protected patch that can be detected,” says Ralston.
During XFP, the x-rays traveling through a solution generate hydroxyl radicals, which then modify the amino acids in the protein that are solvent accessible. They don’t modify the ones that aren’t solvent accessible, which then basically creates a map of all the solvent-accessible areas on a protein. “You can look at huge complexes; the size of the protein doesn’t matter,” says Ralston. “Whereas, in crystallography, trying to crystallize large complexes is very challenging.”
Another benefit of XFP is that it can be done at close to physiological conditions, which gives researchers a more real-life representation of the protein. “XFP is really useful for looking at a protein changing in solution as a function of pH or salt concentration, for instance, or to watch protein-protein interactions and see where they are binding,” says Ralston. “Having this other technique that can give us more information is just really helpful.”
XFP has been a team effort at the ALS, with research scientist Dr. Sayan Gupta, previously employed at NSLS, working out the kinks of the ALS XFP system on the beamline floor. Beamline 5.3.1 scientist Rich Celestre has also been heavily involved, especially with working out a way to focus the high brightness beam at 5.3.1, allowing researchers to utilize microsecond exposures, which opens up the possibility for time-resolved studies on proteins. XFP has also been deployed at Beamline 3.2.1, which offers high flux but not high brightness.