The miniaturization of computing architectures has paved the way for personal hand-held electronic devices (smartphones, tablets, etc.) that feature extraordinary computing power. For such battery-operated devices, keeping the power consumption low while continuing to add features is a major challenge. To address this issue, there is a worldwide research effort dedicated to minimizing the energy required to perform computational operations and to find a successor to silicon integrated circuit (IC) technology, which is approaching fundamental limits. One such architecture, called nanomagnetic logic (NML), employs chains of nanosized magnets instead of transistors. At the ALS, researchers have used a time-resolved x-ray imaging technique with 100-picosecond resolution to directly observe the signal propagation dynamics in these nanomagnetic chains. The technique can assess NML reliability on fast time scales and help determine optimal nanomagnet geometry, spacing, and material.
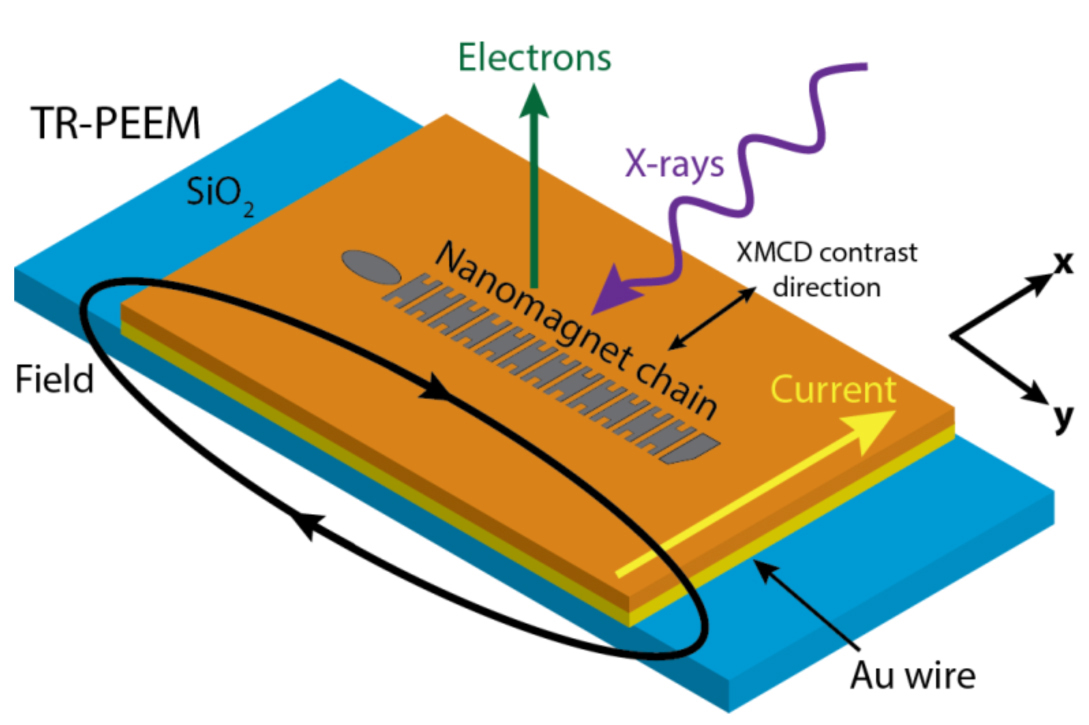
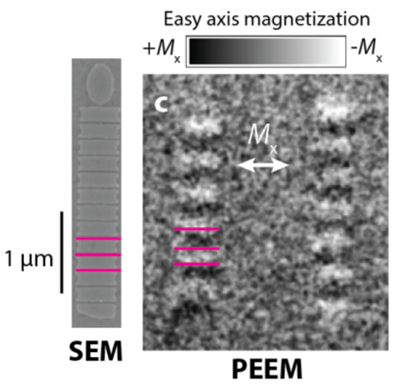
Signal propagation in nanomagnet chains had previously been characterized by static magnetic imaging experiments; however, the mechanisms that determine the final state and their reproducibility over millions of cycles of high-speed operation had yet to be experimentally investigated. Characterizing the time scale of magnetic interactions in this energy-efficient computing architecture provides a critical benchmark comparison to other competing technologies.The energy required to flip a magnet’s orientation (performing a logic function) can be orders of magnitude lower than the operational energy of a single transistor. In NML, the magnetization of single-domain ferromagnetic thin-film islands are coupled by magnetic fields generated from adjacent islands, which impart a preference for neighboring islands to align in antiparallel directions. A chain of these closely spaced nanomagnets propagates binary information from one end to the other sequentially through a series of inversions, performing a function similar to a conventional IC connection but with a potentially lower dissipation energy per switching event.
In this study, researchers characterized NML operation on picosecond time scales and observed, for the first time, the magnetic dynamics within this high-speed regime. They directly imaged digital-signal propagation in permalloy nanomagnet chains using full-field magnetic x-ray transmission microscopy (MTXM) and time-resolved photoemission electron microscopy (TR-PEEM) at the ALS after applying short magnetic-field pulses. These experiments, and accompanying macrospin and micromagnetic simulations, revealed the underlying physics of NML architectures that are repetitively operated at picosecond time scales and identified relevant engineering parameters for optimizing performance and reliability.
Previous studies of NML have used static magnetic imaging (e.g., magnetic force microscopy) to assess the magnetic signal propagation based on the final observed output state. For example, in cases where magnets were perfectly anti-aligned throughout the chain, researchers assumed that perfect dipolar signal propagation occurred.
In this work, the researchers tested those assumptions by directly observing the magnetic signal propagation using an x-ray based imaging technique with 100-ps resolution. First, using MTXM at Beamline 6.1.2, the researchers statistically analyzed the static signal-propagation reliability of nanomagnet chains reset by nanosecond magnetic-field pulses. This identified geometries that optimize signal propagation and verified that short pulses could be reliably employed to perform a time-resolved measurement. After confirming that nanosecond pulses are suitable, the researchers then used TR-PEEM at Beamline 11.0.1.1 to directly image the magnetic signal-propagation dynamics in chains by synchronizing the magnetic-field pulses with pulsed x-rays from the ALS.
Observation of signal propagation by dipolar coupling. (a) Waveform corresponding to the magnetic clock field pulse, measured by detecting transient deflection of the emitted photoelectrons. (b) Averaged time-resolved XMCD-PEEM images of a nanomagnet chain at various time delays from 1 ns to 4 ns from the peak of the magnetic clock pulse shown in (a). The lateral scale in these images is indicated by the 1 µm scale bar. Signal propagation from the third nanomagnet to the eighth nanomagnet driven by dipolar coupling (emphasized in the yellow region) is observed between 1.4 and 1.8 ns. Pink lines distinguish individual nanomagnets in the chain. (c) An interpretation of the switching events observed in part (b). Black and white indicate magnets that have oriented along the easy (x) axis in opposite directions, respectively.
The TR-PEEM data confirms that signal propagation proceeds at a rate of approximately 100 ps per switching event, as predicted previously through computational studies. This time-resolved NML assessment technique introduces the ability to examine the performance of individual nanomagnets during signal propagation, a feature not present in existing quasi-static imaging measurements. Experimentally evaluating the performance of NML chains complements existing time-resolved micromagnetic simulations, offers realistic assessments of nanomagnet designs, and identifies systematic errors and architectural weaknesses.
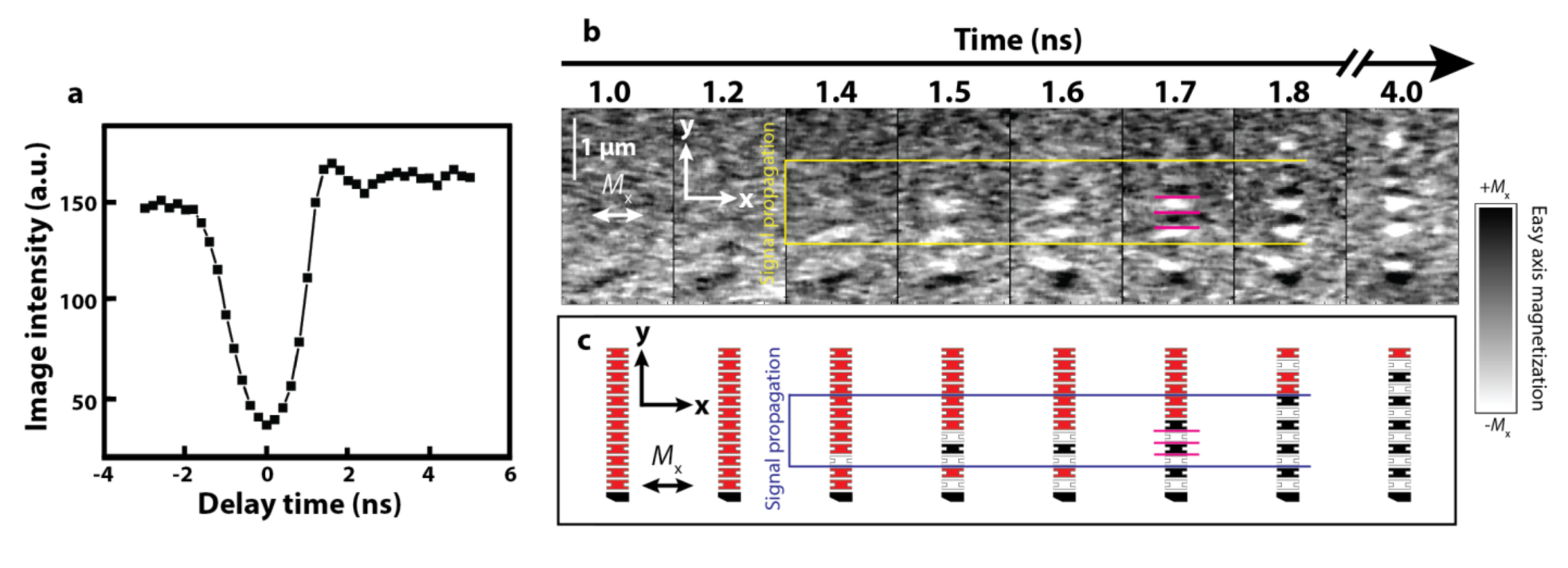
Contact: Jeffrey Bokor
Research conducted by: Z. Gu, M.E. Nowakowski, J. Hong, P. Bennett, and J. Bokor (UC Berkeley); D.B. Carlton and M.T. Alam (Intel Corp.); R. Storz (Thorlabs Inc.); M.-Y. Im (Berkeley Lab and Daegu Gyeongbuk Inst. of Science and Technology, Korea); W. Chao (Berkeley Lab); B. Lambson (iRunway); M.A. Marcus, A. Doran, A. Young, and A. Scholl (ALS); and P. Fischer (Berkeley Lab and UC Santa Cruz).
Research funding: Western Institute of Nanoelectronics; Defense Advanced Research Projects Agency; National Science Foundation; and Ministry of Education, Science and Technology (Korea). Operation of the ALS is supported by the U.S. Department of Energy (DOE), Office of Basic Energy Sciences (BES).
Publication about this research: Z. Gu, M.E. Nowakowski, D.B. Carlton, R. Storz, M.-Y. Im, J. Hong, W. Chao, B. Lambson, P. Bennett, M.T. Alam, M.A. Marcus, A. Doran, A. Young, A. Scholl, P. Fischer, and J. Bokor, “Sub-nanosecond signal propagation in anisotropy-engineered nanomagnetic logic chains,” Nat. Commun. 6, 6466 (2015).
ALS SCIENCE HIGHLIGHT #317