Sometimes, the spins in a magnetic material will form tiny swirls that can move around like particles. The spins themselves stay put—it’s the pattern that moves. These quasiparticles have been dubbed “skyrmions,” after British physicist Tony Skyrme, who described their mathematics in a series of papers in the early 1960s. Now, over 50 years later, scientists are intrigued by the possibility that skyrmions could play a key role in spintronics—electronics that employ spin to carry and maniupulate information. At the ALS, two research groups have recently published separate studies in which soft x-rays reveal how skyrmions react to external fields, laying the foundation for understanding and eventually utilizing these fascinating constructs.
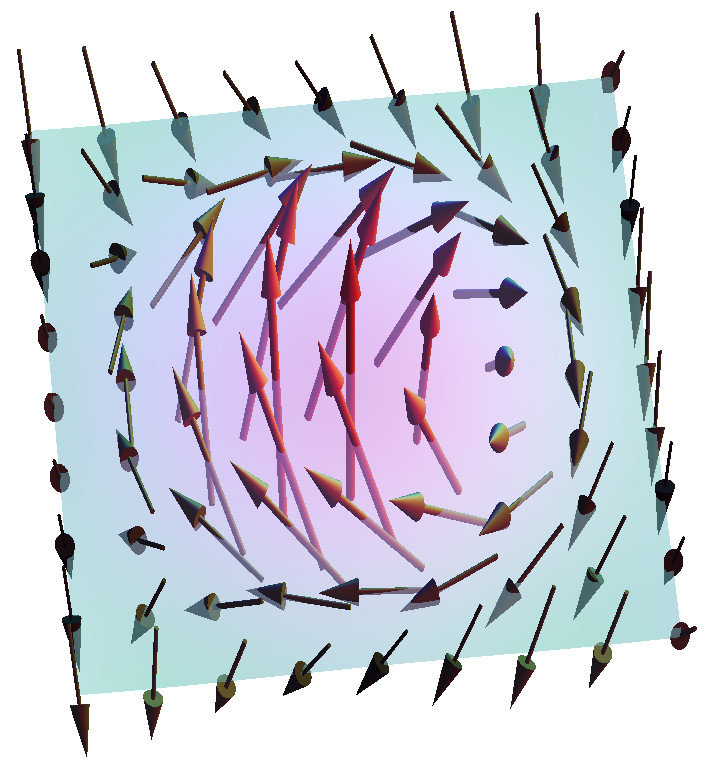
Although skyrmions act like particles (namely, baryons), they are actually magnetic vortices formed from the spins of charged particles. Spin is a quantum property in which the charged particles act like bar magnets rotating about an axis that points either “up” or “down.” The discovery of skyrmions in manganese silicide generated much excitement because their exotic hedgehog-like spin texture is topologically protected and, therefore, extremely stable. Add to this the discovery that skyrmions can be moved coherently over macroscopic distances with a tiny electrical current, and you have a strong spintronic candidate.
A major breakthrough came with the discovery of skyrmions in copper selenite because its magnetic properties can be controlled with an electric field. To achieve this control, however, we must understand how different electron orbitals stabilize the skyrmionic phase. Until now, the copper selenite skyrmions had only been observed with neutron scattering and transmission electron microscopy, techniques that are insensitive to electron orbitals.
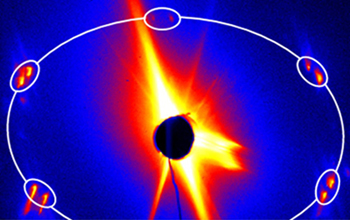
In one skyrmion study done at the ALS, researchers gathered element-specific, orbital-sensitive electronic and magnetic structural information using resonant x-ray scattering at Beamline 12.0.2, optimized for coherent x-ray scattering studies of magnetic materials. They were able to show that although skyrmions act like magnetic particles, their origin in copper selenite is electronic and that temperature can be used to move the skyrmions in either a clockwise or counter-clockwise direction. They also found the unexpected existence of two distinct skyrmion sublattices that rotate with respect to each other, creating a moiré-like pattern. Compared to materials with a simpler magnetic structure, the sublattices provide for an extra degree of freedom to minimize the free energy. This leads to magnetic excitations that can’t exist in materials with a single magnetic lattice structure.
In another study, a second research group created artificial skyrmions in 30-nm-thick cobalt disks on 30-monolayer nickel, all on a copper substrate. This structure created two magnetic regions: the area in/under the cobalt disk (in-plane magnetization), and the surrounding nickel (out-of-plane magnetization). This was important because skyrmions can be characterized by a skyrmion number (N) equal to 0 when the core and surrounding spins are parallel, and 1 when they are antiparallel. By applying various out-of-plane magnetic fields, the researchers were able to control the formation of the two states in this system.
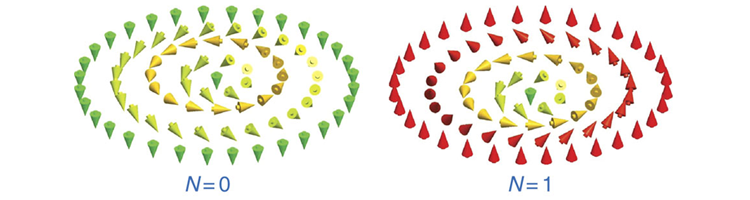
To study the skyrmions’ topological nature, the researchers annihilated the skyrmions by applying an in-plane magnetic pulse that pushed the core out of the disk, converting the vortex into a simple magnetic domain. Photoemission electron microscopy (PEEM) measurements at Beamline 11.0.1.1 revealed that N=1 skyrmions (antiparallel) required a higher critical field for annihilation than N=0 skyrmions (parallel). This distinct behavior for the two states suggests a topological effect of the magnetic skyrmions in the core annihilation process.
The exploration of the world of magnetic skyrmions is just beginning, but it already reveals that these particle-like spin configurations not only hold promise for ultracompact data storage and processing, but they may also open up entirely new areas of study in the emerging field of quantum topology.
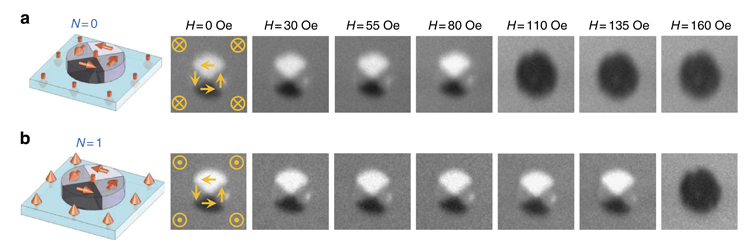
Contacts: Sujoy Roy and Z.Q. Qiu
Research conducted by: M.C. Langner and R.W. Schoenlein (Berkeley Lab); S. Roy, S.K. Mishra, J.C.T. Lee, X.W. Shi, M.A. Hossain, Y.-D. Chuang, A. Doran, M.A. Marcus, A.T. Young, and E. Arenholz (ALS); S. Seki (RIKEN and Japan Science and Technology Agency); Y. Tokura (RIKEN and Univ. of Tokyo); S.D. Kevan (ALS and Univ. of Oregon); J. Li, A. Tan, S. Ma, R.F. Yang, and Z.Q. Qiu (Univ. of California, Berkeley); and K.W. Moon and C. Hwang (Korea Research Institute of Standards and Science).
Research funding: U.S. Department of Energy (DOE), Office of Basic Energy Sciences (BES); National Science Foundation; National Research Foundation of Korea; and China Scholarship Council. Operation of the ALS is supported by DOE BES.
Publications about this research: M.C. Langner, S. Roy, S.K. Mishra, J.C.T. Lee, X.W. Shi, M.A. Hossain, Y.-D. Chuang, S. Seki, Y. Tokura, S.D. Kevan, and R.W. Schoenlein, “Coupled Skyrmion Sublattices in Cu2OSeO3,” Phys. Rev. Lett. 112, 167202 (2014), doi:10.1103/PhysRevLett.112.167202; J. Li, A. Tan, K.W. Moon, A. Doran, M.A. Marcus, A.T. Young, E. Arenholz, S. Ma, R.F. Yang, C. Hwang, and Z.Q. Qiu, “Tailoring the topology of an artificial magnetic skyrmion,” Nat. Commun. 5, 4704 (2014), doi:10.1038/ncomms5704.
ALS SCIENCE HIGHLIGHT #298