SCIENTIFIC ACHIEVEMENT
Scientists have verified exotic electronic properties predicted to emerge in a ferromagnetic material with “kagome” (trihexagonal) lattice symmetry.
SIGNIFICANCE AND IMPACT
The greater understanding of kagome materials afforded by this work helps open up a new path toward goals such as ultralow-power electronic devices and quantum computing.
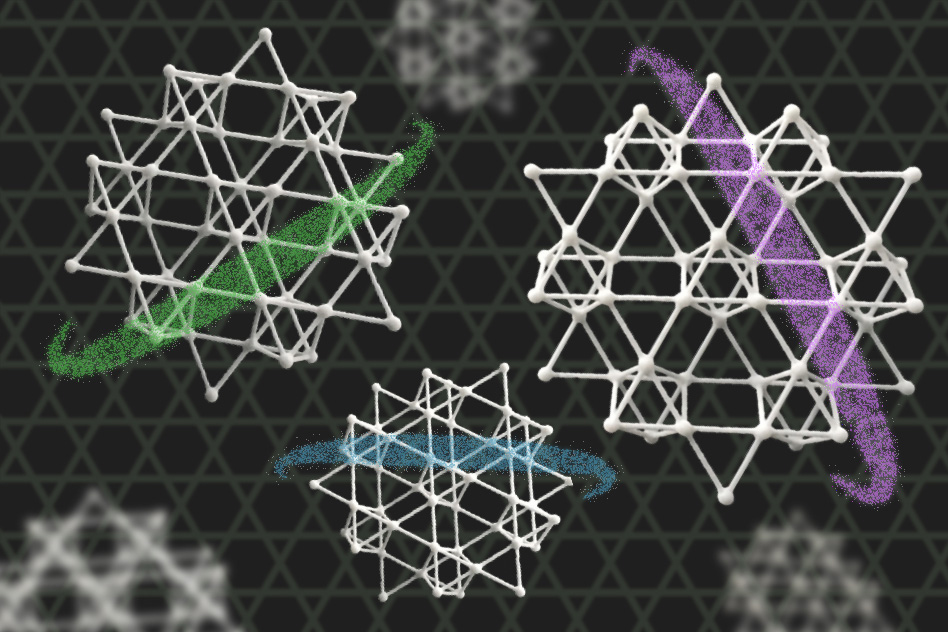
Scientists eye kagome materials

In a “kagome” material, the atoms in a layer form a lattice resembling a traditional Japanese basket-weaving pattern (kago means “basket” and me means “eye,” a reference to the large holes characteristic of this open weave). The quasi-hexagonal symmetry is reminiscent of the hexagonal lattice of graphene, a material well known for its unusual electronic properties.
Recent theoretical developments have suggested that, under some conditions, a kagome material could, like graphene, exhibit a wide range of novel physics. In these phenomena, certain electronic excitations (“massive Dirac fermions”) play a major role. Despite predictions, however, the presence of these electronic modes in kagome compounds has evaded direct experimental observation.
An ideal host
In this work, a team of researchers investigated samples of Fe3Sn2, an alloy of iron and tin. Its unique combination of kagome crystalline symmetry, ferromagnetism, and spin-orbit coupling makes it an ideal host for massive Dirac fermions and the associated Hall effect, in which moving electrons bend into tight, circular paths and flow along edges without losing energy.
When the researchers ran a current across the sample, they indeed observed a temperature-independent, intrinsic Hall effect that persisted above room temperature. Because the kagome network of iron is inherently magnetic, the charges are subject to both the magnetic fields from the iron atoms and a purely quantum-mechanical magnetic force from the lattice, which could lead to perfect conduction, akin to superconductivity, in future generations of materials.
MAESTRO sees a gap
To explore the origins of this Hall response, the researchers mapped the Fe3Sn2 electronic structure using angle-resolved photoemission spectroscopy (ARPES) at ALS Beamlines 7.0.2 (MAESTRO) and 4.0.3 (MERLIN). In particular, the highly efficient measurement process and small spot size available at MAESTRO’s micro-ARPES chamber greatly benefited these experiments, which were complicated by the fast aging of the sample surfaces as well as by small sample sizes.
At MAESTRO, the researchers were able to collect high-quality band-structure data, allowing them to visualize, for the first time, the double-Dirac-cone structure corresponding to Dirac fermions. In addition, by looking at the data in various ways (i.e, taking the second derivative of the raw ARPES data as well as stacking energy-distribution curves), the researchers were able to resolve a 30 meV gap between the Dirac cones, a characteristic of the massive Dirac fermions that generate Hall conductivity.
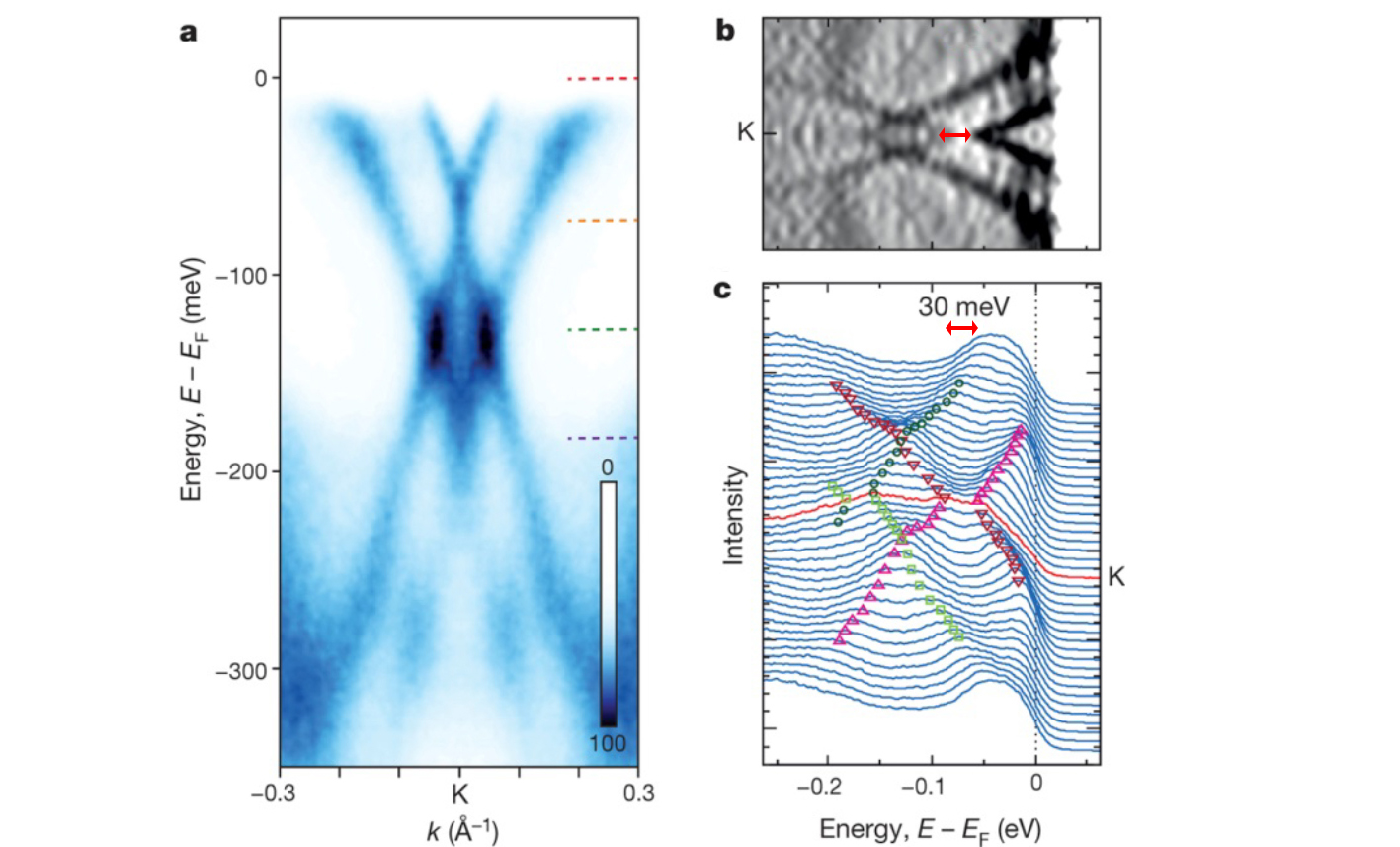
The topology of kagome
Massive Dirac fermions act as a source of “Berry curvature” (a local manifestation of the geometric properties of electronic wavefunctions), which in turn controls the “topological” properties of the sample. Nontrivial wavefunction topologies induce exotic electronic properties such as dissipationless electric currents at the physical edges of a crystal, and materials with such topologically protected states are poised to enable new research directions applicable to low-power electronic devices.
In the future, the researchers plan to investigate the electronic structures of various kagome compounds with different dimensionality, layer stacking configurations, spin properties, and spin-orbit coupling strengths. Such materials, if they can be synthesized, could be used to explore not only devices with zero energy loss, but applications in quantum computing as well.
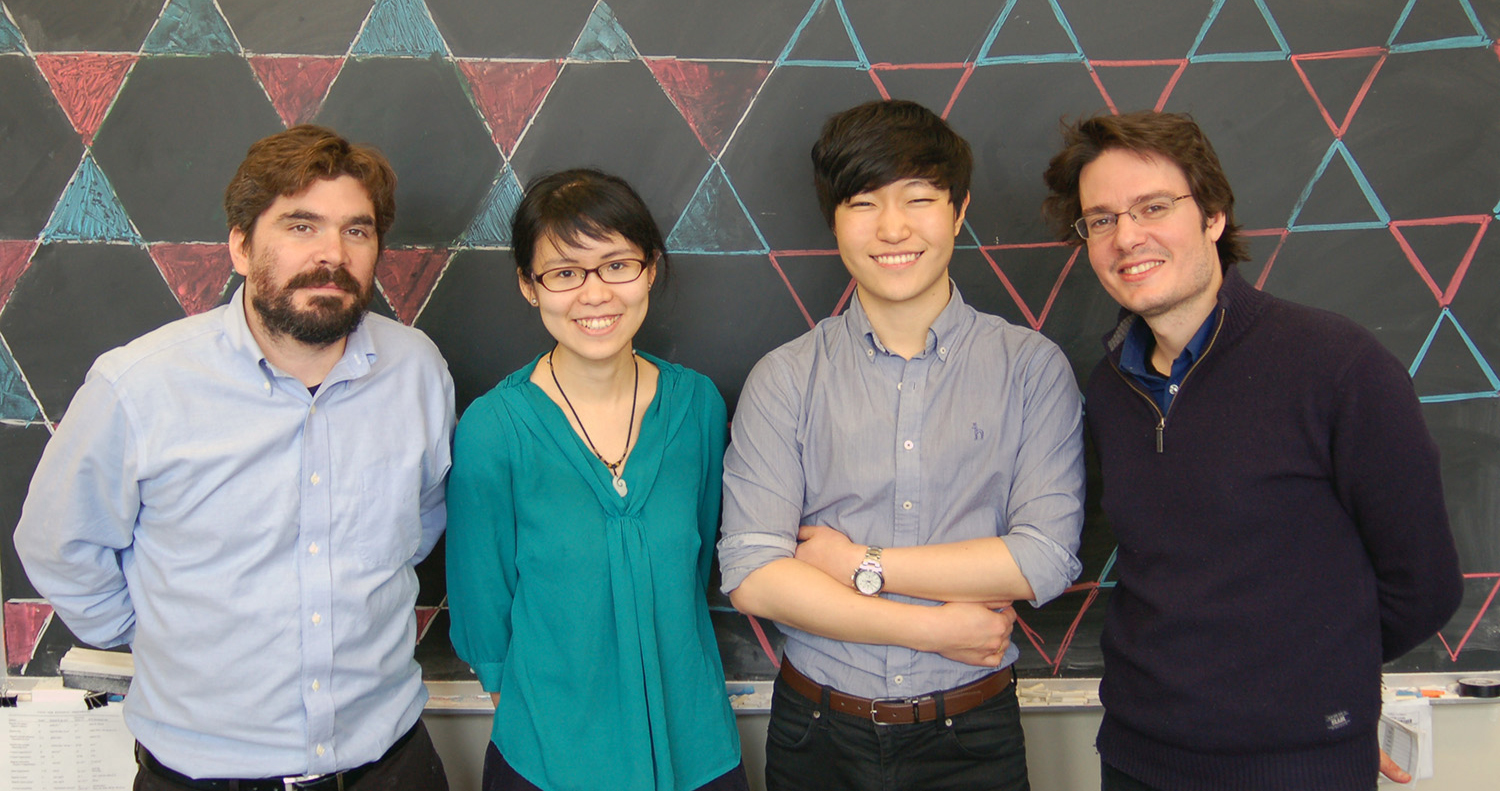
Contacts: Min Gu Kang, Riccardo Comin, and Joe Checkelsky.
Researchers: L. Ye, M.G. Kang, J. Liu, C.R. Wicker, T. Suzuki, L. Fu, R. Comin, and J.G. Checkelsky (Massachusetts Institute of Technology); F. von Cube and D.C. Bell (Harvard University); and C. Jozwiak, A. Bostwick, and E. Rotenberg (ALS).
Funding: Gordon and Betty Moore Foundation, National Science Foundation, Tsinghua Education Foundation, Samsung Foundation of Culture, and the State of Florida. Operation of the ALS is supported by the U.S. Department of Energy, Office of Science, Basic Energy Sciences Program (DOE BES).
Publication: L. Ye, M.G. Kang, J. Liu, F. von Cube, C.R. Wicker, T. Suzuki, C. Jozwiak, A. Bostwick, E. Rotenberg, D.C. Bell, L. Fu, R. Comin, and J.G. Checkelsky, “Massive Dirac fermions in a ferromagnetic kagome metal,” Nature 555, 638 (2018), doi: 10.1038/nature25987.
Adapted from the MIT press release, “Physicists discover new quantum electronic material.”
ALS SCIENCE HIGHLIGHT #376