For extreme applications such as nuclear fusion reactors and high-temperature jet engines, scientists are experimenting with “high-entropy” alloys that consist of many metals mixed together in equal proportions. In this work, a team led by researchers from Pacific Northwest National Laboratory (PNNL) and North Carolina State University (NCSU) have begun to unravel how these materials degrade under high-temperature oxidative environments.
“One common way that materials degrade is oxidation—materials interact with oxygen and break down,” said Bharat Gwalani, corresponding author of a paper on the work and an assistant professor of materials science and engineering at NCSU. “This work sheds light on exactly how oxidation takes place in complex alloys and will inform both the development of future alloys and how we can use those alloys in real-world applications.”
The researchers studied a high-entropy alloy with equal amounts of the metals cobalt, chromium, iron, nickel, and manganese (CoCrFeNiMn, also called the Cantor alloy) using atom probe tomography, electron microscopy, grazing-incidence wide-angle x-ray scattering at the National Synchrotron Light Source II (NSLS-II), and x-ray absorption near-edge structure (XANES) at Advanced Light Source (ALS) Beamline 7.3.1. The ALS XANES measurements captured interactions between the elements, indicated by peak shifts—or lack thereof—before and after oxidation.
“Correlating x-ray spectroscopy results from the ALS with in situ atom probe tomography results from PNNL in this way can be very powerful for analyzing the degradation mechanisms of materials in many different types of extreme environments,” said Arun Devaraj, a materials scientist at PNNL and also a corresponding author on the paper.
The researchers also applied a thermodynamical model called the Preferential Interactivity Parameter (PIP), developed by colleagues at NCSU, which evaluates parameters such as cohesive energy density, electronegativity, and atomic radius to realistically assess elements’ oxidation tendencies in diverse environments. Comparison of the PIP outputs with experimental results identified a correlation between the two.
Overall, the results showed that the fast-oxidizing element chromium controlled early oxidation, stabilizing the inner oxide layer, while the slower-oxidizing elements manganese, iron, and cobalt diffused outward and dominated the outer layer. Nickel maintained crystalline stability of the base alloy, forming a layer beneath the oxidation.
With this work, the researchers aim to provide “a new template to design microstructures that can fill in the gaps between room-temperature properties to high-temperature properties and between cheaper material to expensive material to create cheaper and stronger materials for higher-temperature applications,” said Gwalani.
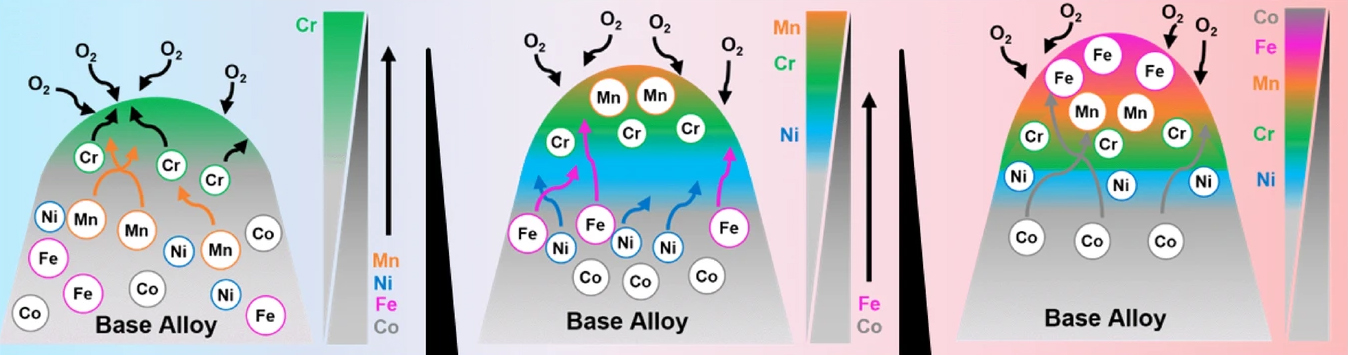
B. Gwalani, A. Martin, E. Kautz, B. Guo, S.V. Lambeets, M. Olszta, A.K. Battu, A. Malakar, F. Yang, J.-H. Guo, T. Suntharampillai, R. Li, A. Amassian, M. Thuo, and A. Devaraj, “Mechanistic Understanding of Speciated Oxide Growth in High Entropy Alloys,” Nat. Commun. 15, 5026 (2024), doi:10.1038/s41467-024-49243-8.
Adapted from articles published by Pacific Northwest National Laboratory (“Metal Alloys that Can Take the Heat”) and NCSU (“Complex Alloys Reacting with Oxygen Paves Way for Better Alloys”).