SCIENTIFIC ACHIEVEMENT
Researchers designed and fabricated a nanomagnet array in which competing (“frustrated”) magnetic interactions can be directly tuned, enabling detailed studies of the system’s properties.
SIGNIFICANCE AND IMPACT
Frustrated interactions are key to a wide range of phenomena, from protein folding and magnetic memory to fundamental studies of emergent exotic states.

Geometrical frustration and “spin ice”
When bar magnets are brought together, opposite poles will attract and like poles will repel, and the magnets will arrange themselves accordingly, to minimize energy. However, if the magnets are constrained to a lattice structure where each one has just two possible orientations, some magnets could end up geometrically “frustrated,” with neither orientation being lower in energy than the other. The system becomes what’s known as a “spin ice,” analogous to water ice, which retains intrinsic randomness (residual entropy) even at absolute zero.
Systems incorporating geometrical frustration are fascinating because their hard-to-predict behavior is key to a wide range of phenomena, from protein folding and magnetic memory to the emergence of exotic states of matter. For example, the emergence of magnetic monopole–like excitations in spin ice raises the intriguing possibility of “magnetic-charge” circuitry based on currents of magnetic monopole excitations.
Artificial spin ice systems: the trident
Although real three-dimensional examples of spin ice exist, advanced fabrication techniques enable researchers to design two-dimensional versions that provide greater control over key parameters and are simpler to study. Over the past 12 years, square- and hexagon-based artificial spin ice systems have been investigated extensively, but new geometries exhibiting a higher degree of spin frustration at low temperatures was needed. In this work, researchers came up with an interesting new design: the dipolar trident lattice.
Taking advantage of state-of-the-art electron-beam lithography available at the Molecular Foundry, the researchers created a pattern consisting of tridents that are tiled perpendicularly to each other. Because the nanomagnets couple via dipolar magnetic fields, the system is called a dipolar trident lattice. With full control of the lattice parameters—nanomagnet length (L), nanomagnet width (W), the distance between nanomagnets within a trident (a), and the distance between trident blocks (b)—the researchers sought to tune the degree of geometrical frustration in the system.
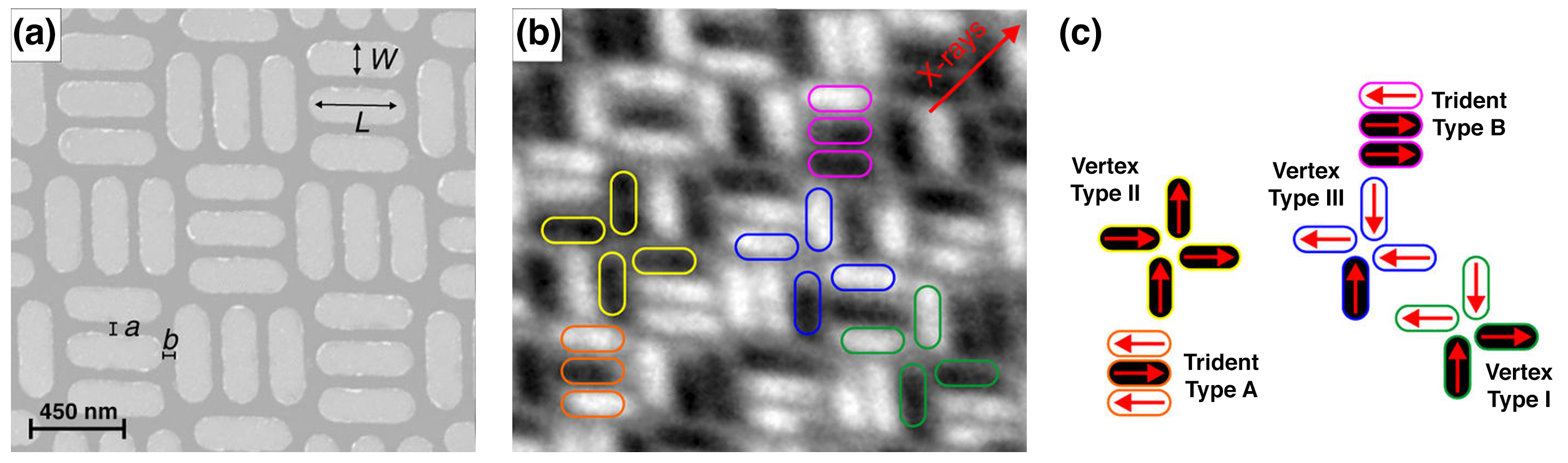
ALS Beamline 11.0.1.1 reveals frustration
The lattices were visualized at the photoemission electron microscope (PEEM3) at ALS Beamline 11.0.1.1, using x-ray magnetic circular dichroism (XMCD) at the Fe L3 edge to show magnetic contrast. The degree of frustration in the system was quantified by looking at the mix of vertex and trident types for different values of the ratio b/a. The researchers found that a phase with no clear preference for any vertex or trident type (i.e. a disordered phase) occurred at a b/a value of 1.5.
To explore whether this behavior persists at low temperature, the researchers repeated the experiment (with slightly different lattice parameters) at T = 150 K. Although long-range order was observed for b/a values of 1 and 2, the array remained disordered for b/a = 1.5. Magnetic scattering patterns calculated from the XMCD data provided strong evidence that the researchers were indeed able to tune the lattice between two long-range ordered phases and a highly disordered intermediate phase, down to 150 K.
However, the true ground state of the lattice, where maximum spin frustration is achieved, is still an open question. It will require future work involving experimental developments—such as the improvements in light-source quality associated with the ALS Upgrade (ALS-U) project—as well as advances in theory.

Contact: Alan Farhan
Researchers: A. Farhan and A. Scholl (ALS); C.F. Petersen, Q.H. Qin, M.J. Alava, and S. Van Dijken (Aalto University, Finland); S. Dhuey (Berkeley Lab); L. Anghinolfi (Università di Genova, Italy); M. Saccone (University of California, Santa Cruz); S. Velten (Berkeley Lab and Universität Hamburg, Germany); C. Wuth (Berkeley Lab and Daegu Gyeongbuk Institute of Science and Technology, Republic of Korea); S. Gliga (University of Glasgow, UK); and P. Mellado (Adolfo Ibáñez University, Chile).
Funding: Swiss National Science Foundation, Academy of Finland, Aalto University, Horizon 2020 Research and Innovation Programme (European Union). Operation of the ALS is supported by the U.S. Department of Energy, Office of Science, Basic Energy Sciences Program.
Publication: A. Farhan, C.F. Petersen, S. Dhuey, L. Anghinolfi, Q.H. Qin, M. Saccone, S. Velten, C. Wuth, S. Gliga, P. Mellado, M.J. Alava, A. Scholl, and S. Van Dijken, “Nanoscale control of competing interactions and geometrical frustration in a dipolar trident lattice,” Nature Communications 8, 995 (2017), doi:10.1038/s41467-017-01238-4.
ALS SCIENCE HIGHLIGHT #368