SCIENTIFIC ACHIEVEMENT
Researchers working at the Advanced Light Source (ALS) showed that hafnium oxide surprisingly exhibits enhanced ferroelectricity (reversible electric polarization) as it gets thinner.
SIGNIFICANCE AND IMPACT
The work shifts the focus of ferroelectric studies from more complex, problematic compounds to a simpler class of materials and opens the door to novel ultrasmall, energy-efficient electronics.
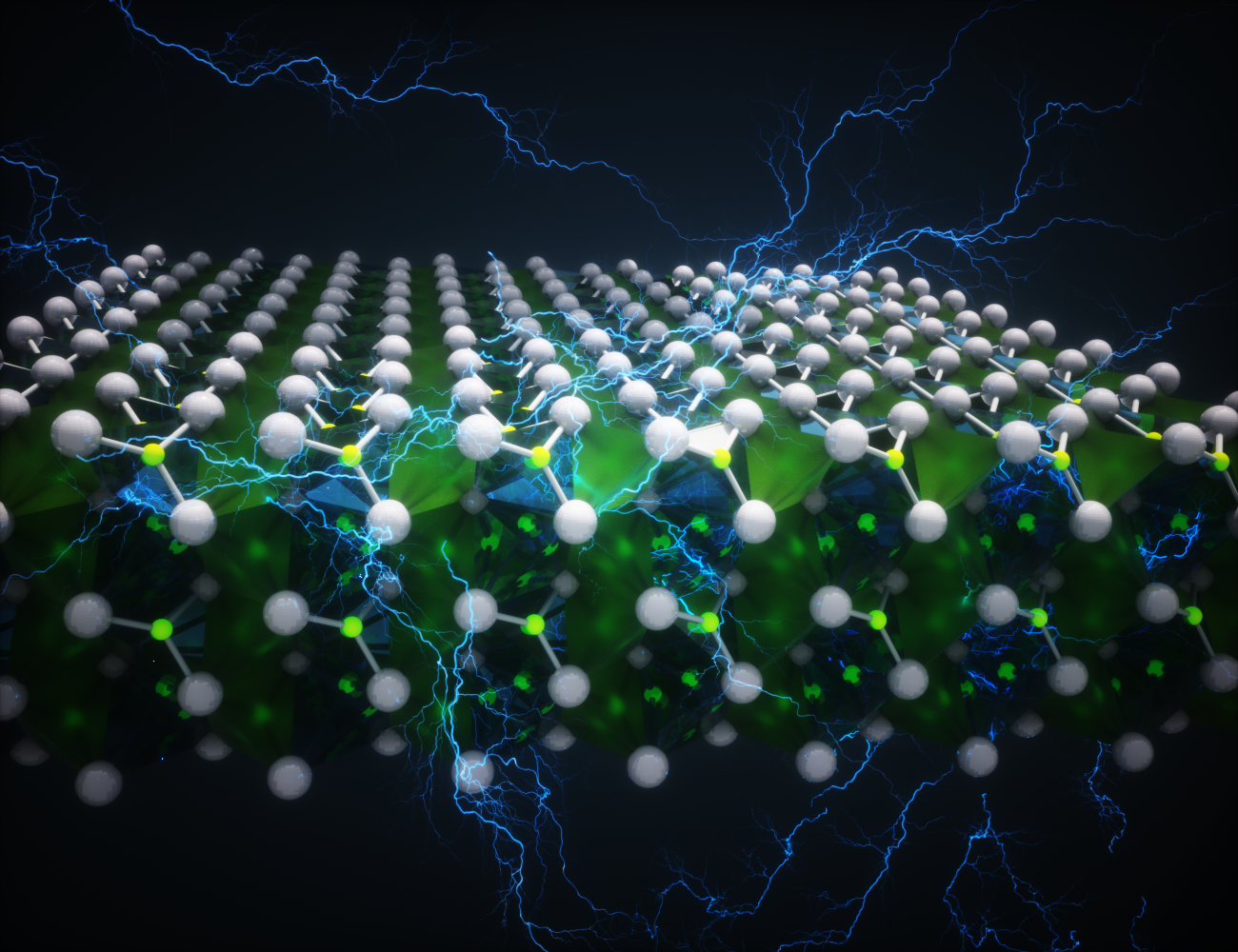
Ferroelectric lower limit?
Distortions in the atomic geometries of certain materials can lead to ferroelectricity—the presence of electric dipoles (charge separations) with switchable polarizations. The ability to control this polarization with an external voltage offers great promise for ultralow-power microprocessors and nonvolatile memory.
As electronic devices become smaller, however, the materials used to store and manipulate electronic data are being pushed to low-dimensional extremes. Properties that function reliably in bulk materials often diminish in ultrathin films just a few atomic units thick. Therefore, exploring the critical thickness limit in “polar” materials (i.e., materials having spontaneous electric polarization) is not only a fundamental issue for nanoscale ferroelectric research, it also has extensive implications for the future of high-density ferroelectric-based electronics.
Tinkering with hafnium oxide
Past studies of ferroelectric size effects primarily focused on perovskites, complex oxides that have so far proven to be incompatible with today’s silicon-based technologies. In this work, researchers instead investigated zirconium-doped hafnium oxide (HfO2) on silicon. HfO2 is already used in today’s computer chips and, as a binary oxide, it’s simpler than complex-oxide perovskites. However, in its bulk form, HfO2 stabilizes in a centrosymmetric structure (i.e., no off-center atomic displacement), inconsistent with ferroelectricity.
With some tinkering (annealing and capping with a thick metal), the researchers were able to synthesize ferroelectric HfO2 and stabilize it down to a thickness of 1 nm. The material’s structural characteristics were confirmed using transmission electron microscopy at Berkeley Lab’s Molecular Foundry and x-ray diffraction at the Advanced Photon Source and Stanford Synchrotron Radiation Lightsource.
Spectral signatures of distortion
At the ALS, the researchers employed x-ray absorption spectroscopy (XAS) and x-ray linear dichroism (XLD) at Beamline 4.0.2, as well as photoemission electron microscopy (PEEM) at Beamline 11.0.1.1, to explore the structural and electronic origins of the ferroelectricity in ultrathin HfO2.
XAS is uniquely tailored to probe the spectroscopic signatures of structurally distorted polar (ferroelectric) and nonpolar phases as sample thickness decreases. In addition, PEEM’s ability to generate spatially resolved XLD contrast at selected energies clearly demonstrated the appearance of a polarization texture at a key energy identified by the XAS data. Element-specific XLD measurements quantified the degree of electronic-orbital polarization as a gauge of structural distortion.

A trending surprise
The results demonstrate that, not only is ferroelectricity in Zr-doped HfO2 sustained to the single-nanometer limit (equivalent to two unit cells of HfO2), but the ferroelectric polar distortions are unexpectedly amplified as the thickness is reduced—a trend that does not occur in more conventional perovskite counterparts.
The researchers’ future plans include pushing the ferroelectric size limit even further, to the subnanometer scale, and implementing this silicon-compatible system in ferroelectric tunnel junctions, a promising nonvolatile-memory candidate. In the longer term, atomic-scale heterostructures utilizing ultrathin ferroelectric order on silicon could be used to stabilize negative capacitance, an emergent physical phenomenon that is promising for ultralow-power transistors.
More generally, this work shifts the search for the fundamental limits of ferroelectricity to simpler transition-metal oxide systems—that is, from perovskite-derived complex oxides to fluorite-structure binary oxides—in which “reverse” size effects counterintuitively enhance ferroelectricity in the ultrathin regime.
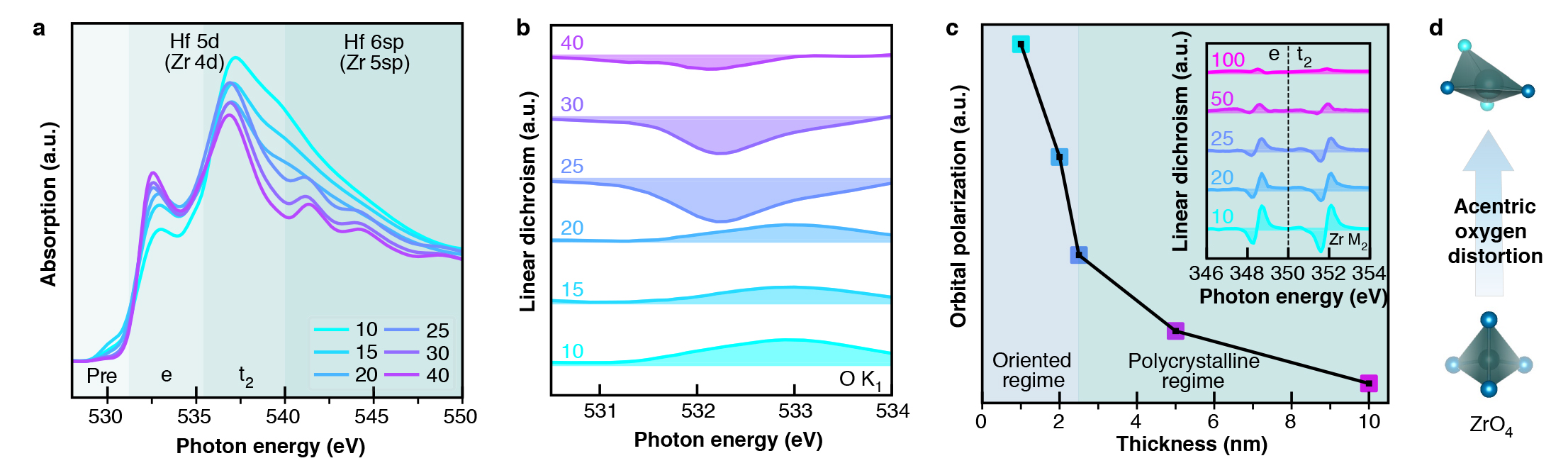
Contacts: Suraj Cheema and Sayeef Salahuddin
Researchers: S.S. Cheema, D. Kwon, N. Shanker, J. Xiao, A. Datar, M.R. McCarter, C.R. Serrao, A.K. Yadav, G. Karbasian, C.‑H. Hsu, A.J. Tan, L.‑C. Wang, V. Thakare, X. Zhang, C. Hu, and R. Ramesh (UC Berkeley); R. dos Reis, S.‑L. Hsu, and J. Ciston (Berkeley Lab); H. Zhang, R. Wagner, and R. Proksch (Oxford Instruments Asylum Research); A. Mehta (SLAC National Accelerator Laboratory); E. Karapetrova (Argonne National Laboratory); R.V. Chopdekar, P. Shafer, and E. Arenholz (ALS); and S. Salahuddin (UC Berkeley and Berkeley Lab).
Funding: Berkeley Center for Negative Capacitance Transistors; Joint University Microelectronics Program (a Semiconductor Research Corporation program sponsored by DARPA); the DARPA Technologies for Mixed-mode Ultra Scaled Integrated Circuits (T-MUSIC) program; and the University of California Multicampus Research Programs and Initiatives project. Operation of the ALS is supported by the U.S. Department of Energy, Office of Science, Basic Energy Sciences program (DOE BES).
Publication: S.S. Cheema, D. Kwon, N. Shanker, R. dos Reis, S.‑L. Hsu, J. Xiao, H. Zhang, R. Wagner, A. Datar, M.R. McCarter, C.R. Serrao, A.K. Yadav, G. Karbasian, C.‑H. Hsu, A.J. Tan, L.‑C. Wang, V. Thakare, X. Zhang, A. Mehta, E. Karapetrova, R.V. Chopdekar, P. Shafer, E. Arenholz, C. Hu, R. Proksch, R. Ramesh, J. Ciston, and S. Salahuddin, “Enhanced ferroelectricity in ultrathin films grown directly on silicon,” Nature 580, 478 (2020), doi:10.1038/s41586-020-2208-x.
ALS SCIENCE HIGHLIGHT #421