As crystallographers seek to tackle ever more challenging problems in microbiology, getting the maximum amount of data out of each delicate and expensive crystal is extremely difficult, and requires the highest-precision tools to monitor and adjust the x-ray exposure. Working at the ALS, Berkeley Center for Structural Biology’s Senior Scientific Engineering Associate Diane Bryant and Staff Scientist Simon Morton recently developed a cutting-edge beamstop that combines two essential crystallographic functions—capturing the damaging portion of the beam while simultaneously monitoring its intensity—into a single miniaturized package. The Compact Dynamic Beamstop (CDBS) technology has been licensed and made commercially available by MiTeGen and is also a finalist for an R&D 100 Award.
“For a long time people have known we’ve needed something like this,” says Morton. “It needs to be small, simple and robust, and have a high level of sensitivity, but no one has quite managed to do all of those things in one device.”
“The challenge with other devices that have been developed is that they’re hard to reproduce on a larger scale,” he adds. “There’s a ‘maximum tolerable size’ where at some point it starts to impact the experiment.”
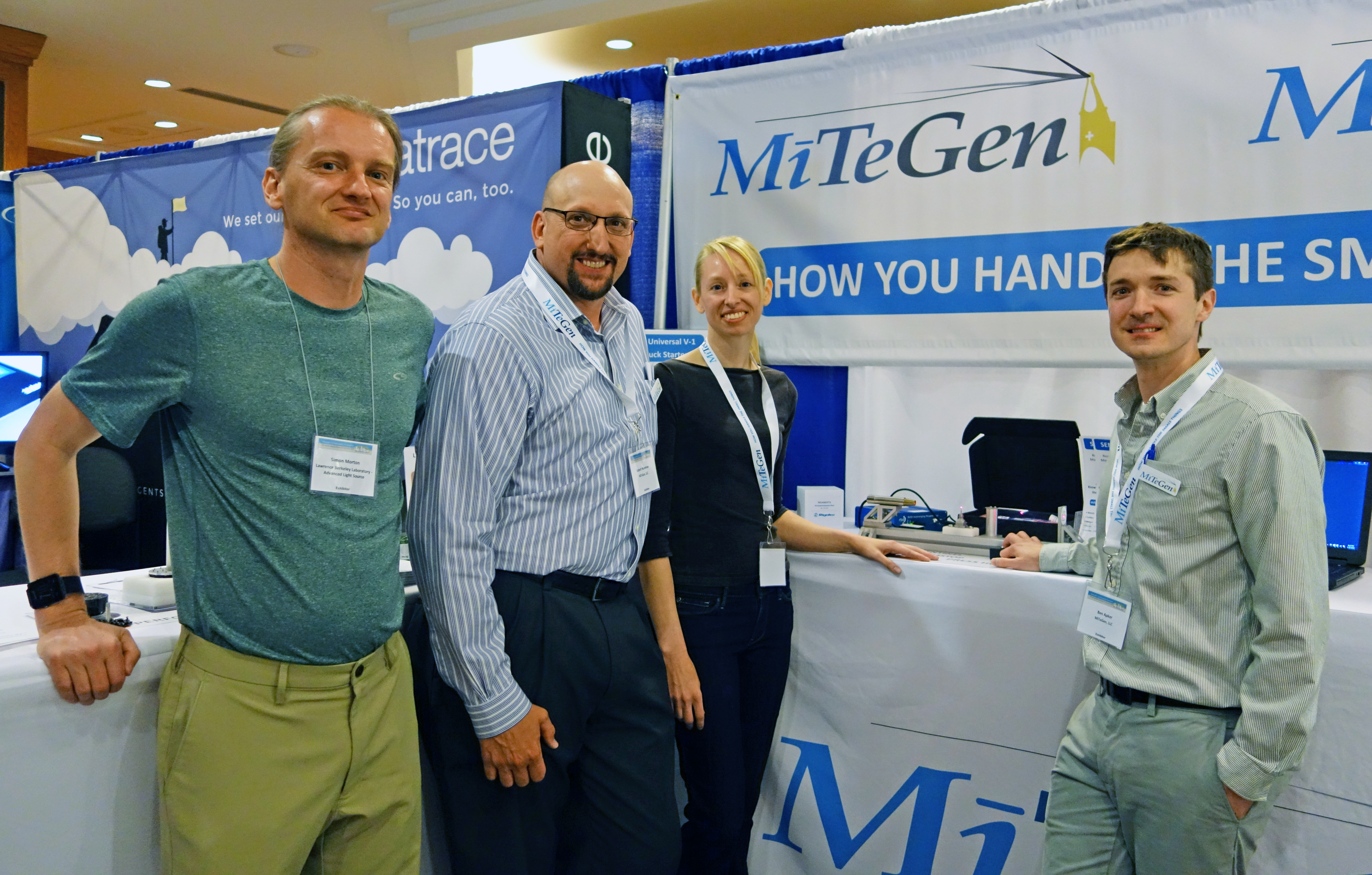
Over the past 20 years, protein crystallography has become a critical tool in the development of groundbreaking new therapies for cancer, HIV, TB, Ebola, and a host of other life-threatening diseases. Understanding the protein structure of these diseases helps scientists develop better, more targeted treatments. Protein crystallography research involves small crystals of high-purity proteins being exposed to very intense, tightly focused x-ray beams that probe their molecular structure and biological function. The success of these experiments often hinges on the use of extremely sensitive x-ray detectors that give scientists access to real-time monitoring information about the x-ray dose their samples are exposed to.
A tiny beamstop, made of a material with good x-ray blocking ability, is necessary to prevent the incoming x-ray beam from directly striking the detector. This beamstop must be as small as possible so as not to block the critical x-rays emitted from the protein sample that carry vital data. The challenge is creating a device integrated into a beamstop that can accurately measure and monitor crystallography experiments without damaging them or interfering with the x-ray beams. The beamstop device has to be very small, very accurate, and if it is to be commercially reproducible, quick and relatively inexpensive to manufacture. “Some of these crystals are very fragile and very expensive, and they get damaged very easily,” says Morton. “Each one has a finite amount of radiation it can take and a finite amount of data that can be extracted in that time. Too much radiation too early and you’ll fry it before you can get all your data. On the other hand, if you don’t put in enough photons you’re leaving data on the table.”
Bryant and Morton began collaborating on the project about three years ago, when Morton approached Bryant with two possible ideas for making an intensity monitoring beamstop. The potential challenge of making such a useful device on such a small scale was irresistible to Bryant, and she proceeded down the path that seemed most likely to succeed. After multiple rounds of refinement, she’s now on her 12th generation of the prototype. Last year Bryant and Morton approached MiTeGen, a company that engineers and produces tools for crystallography to academic, medical, and industrial labs, about licensing the technology they’d developed. MiTeGen saw the potential in the CDBS and recently launched a product based on the technology they licensed from the ALS, called the Sentinel Beamstop System.
“The work done by the team at LBNL has resulted in a great tool for monitoring x-ray diffraction experiments,” says Robert Newman, CEO of MiTeGen. “The beamstop device will enable researchers to obtain real-time information about the intensity of the x-rays hitting their samples.”
“We recently launched the product at the end of July, and we are already seeing great interest from synchrotron facilities all around the world,” says Benjamin Apker, director of business development at MiTeGen. “The response has proven the device addresses a clear need in the x-ray diffraction research community.”
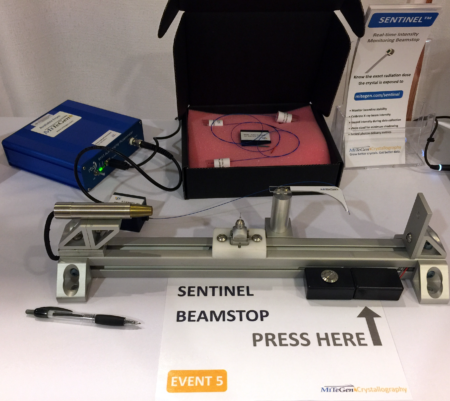
At the heart of the CDBS is an array of tiny, precision-cut scintillator crystals. These crystals convert the intense beam of x-rays into visible light. They are tightly packed and precisely positioned within an enveloping micromachined sleeve only 1 mm in diameter. The sleeve preserves the high-precision alignment of the crystals and helps capture the light emerging from them. As an integral part of the beamstop assembly it also absorbs any residual x-rays that might otherwise pass through or around the crystals and reach the detector. The visible light is then transmitted through a bundle of optical fibers which emerges from the side of the sleeve. The fibers carry the light to electronics where they are converted into an electrical current signal. They also support and precisely position the device in the 100 µm x-ray beam to within a tolerance of a few microns. The tiny diameter of the sensor package—combined with the use of amorphous, low cross-section optical fibers as the sole mechanical support and alignment structure—delivers a diagnostic device with unrivaled sensitivity and small volume; the CDBS is orders of magnitude smaller in volume than any other alternative.
Bryant and Morton are optimistic about how this technology will affect future research at the ALS. “We have this capability and it’s still new enough that we don’t yet know all the possible ways in which it can be used,” says Bryant. “We know that it will be very useful for figuring out radiation doses to samples, but we’ll be interested in seeing how users will implement it for their particular experiments.”