Researchers recently uncovered the first step in the process that transforms gas-phase molecules into solid particles like soot and other carbon-based compounds. It’s a discovery that could help combustion chemists make more efficient, less polluting fuels and help materials scientists fine-tune their carbon nanotubes and graphene sheets for faster, smaller electronics. In addition, the results could have implications for the burgeoning field of astrochemistry, potentially establishing the chemical process for how gaseous outflows from stars turn into carbon-based matter in space.
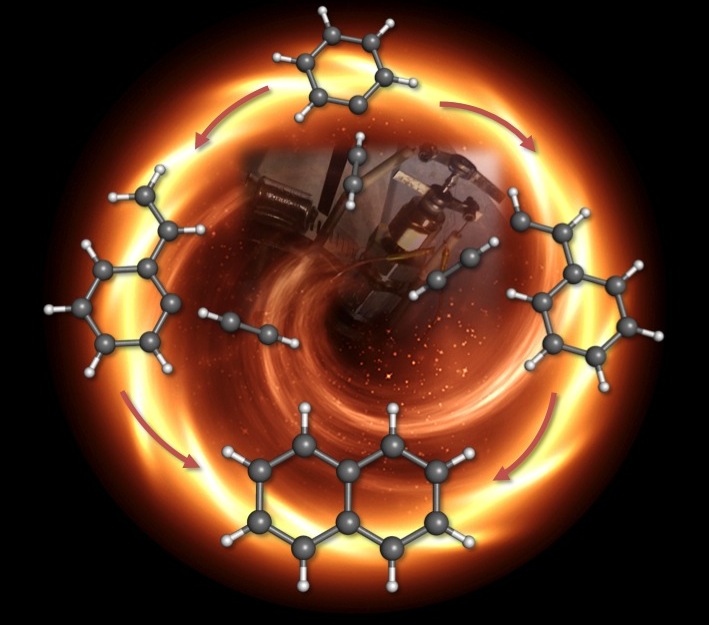
For more than 30 years, scientists have developed computational models of combustion to explain how gas molecules form soot, but now scientists have data to confirm one long-standing theory in particular. The fundamental chemistry discovery could be used to find or design fuels that burn cleaner and don’t produce as much soot.
The combustion process produces fumes and particulates like soot, a visible macromolecule made up of sheets of carbon. Theoretically, there are hundreds of different ways molecules can combine to create these dirty emissions. But there has been one popular class of mechanisms that outlines possible early steps for bond making and bond breaking during combustion: hydrogen abstraction-acetylene addition (HACA).
In one version of HACA, during the high-temperature, high-pressure environment of combustion, a simple ring of six carbon and six hydrogen atoms, called benzene, would lose one of its hydrogen atoms, allowing another two-carbon molecule called acetylene, to attach to the ring, giving it a kind of tail. Then the acetylene tail would lose one of its hydrogen atoms so another acetylene could link up in, doubling the carbon atoms in the tail to four.
Next, the tail would curl around and attach to the original ring, creating a double-ring structure called naphthalene. Link by link, ring by ring, these molecules would continue to grow in an unwieldy, crumpled way until they became the macromolecules that we recognize as soot.
For almost half a century, polycyclic aromatic hydrocarbons (PAHs) have been proposed to play a key role in the astrochemical evolution of the interstellar medium (ISM) and in the chemistry of combustion systems. However, even the most fundamental reaction mechanism assumed to lead to the simplest PAH naphthalene—the HACA mechanism—has historically eluded experimental observation.
To test the first step of the theoretical HACA mechanism, scientists used a beamline at the ALS specifically outfitted to study chemical dynamics. The ALS produces numerous photons over a wide range of energies, allowing researchers to probe a variety of molecules produced in this chemical reaction with specialized mass spectrometry analysis.
Unique to this experimental setup, researchers used a so-called hot nozzle, which recreates combustion environment in terms of pressure and temperature. Scientists started with a gaseous mix of nitrosobenzene (a benzene ring with a molecule of nitrogen and oxygen attached) and acetylene, and pumped it through a heated tube at a pressure of about 300 torr and a temperature of about 750 degrees Celsius. The molecules that came out the other end were immediately skimmed into a mass spectrometer that made use of the synchrotron light for analysis.
The researchers found two molecules predominantly emerged from the process. The more abundant was the carbon ring with a short acetylene tail on it, called phenylacetylene. But they also saw evidence for the double ring, naphthalene. These results effectively rule out one HACA mechanism—that a carbon ring would gain two separate tails and those tails would bond to form the double ring—and confirm the most popular HACA mechanism where a long tail curls around to form naphthalene.
The next step will be to unravel the pathways to more complex systems. Further experiments will investigate these follow-up mechanisms. Researchers will add infrared spectroscopy to their analysis in order to catch the variety of molecules that form during these next phases of combustion.
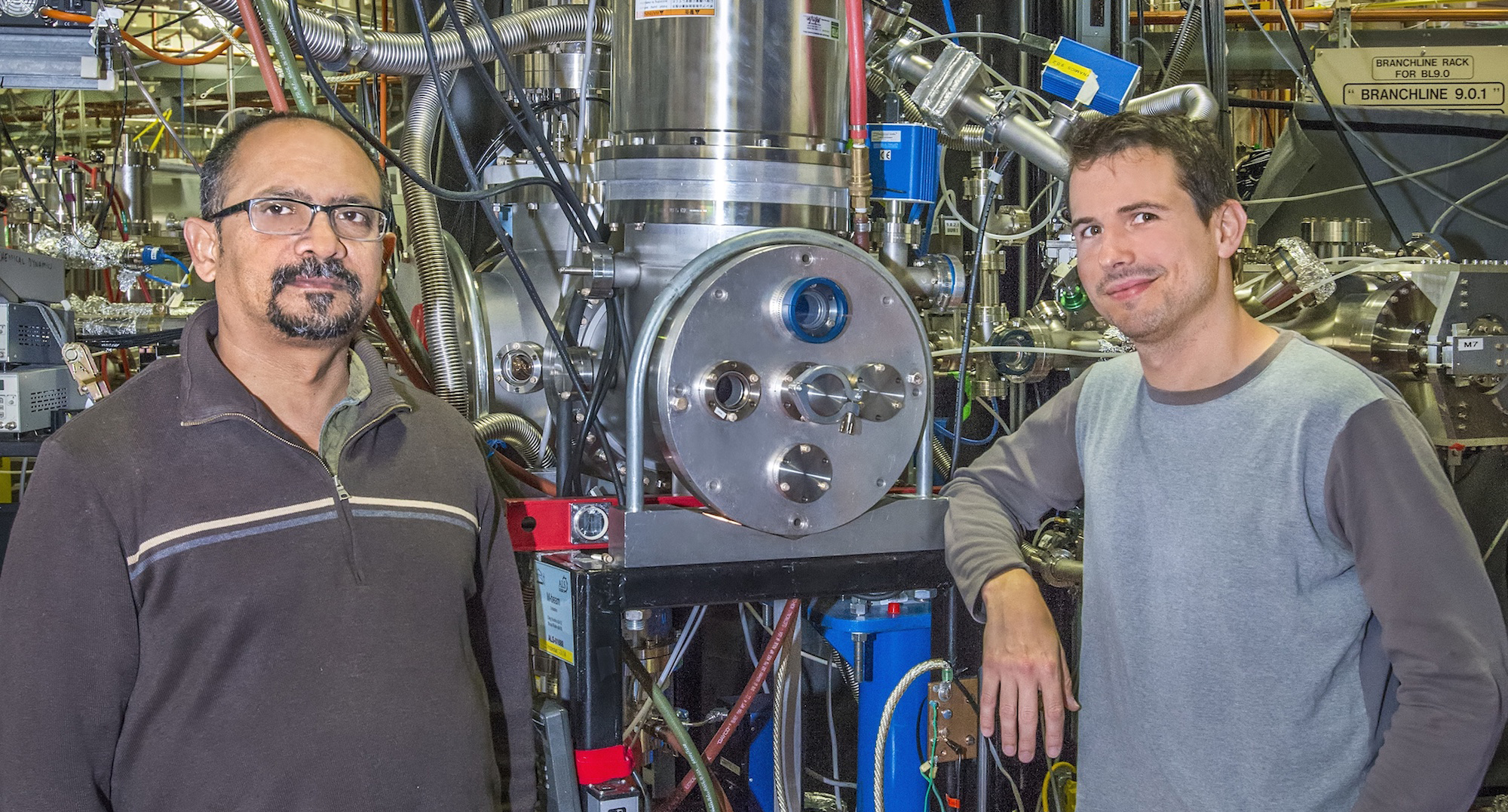
Contact: Ralf Kaiser
Research conducted by: D.S.N. Parker and R.I. Kaiser (University of Hawaii at Manoa ), T.P. Troy and M. Ahmed (Lawrence Berkeley National Laboratory)
Research funding: US Department of Energy, Basic Energy Sciences. Operation of the ALS is supported by the U.S. Department of Energy, Office of Basic Energy Sciences.
Publication about this research: D.S.N. Parker, R.I. Kaiser, T.P. Troy, and M. Ahmed, “Hydrogen Abstraction/Acetylene Addition Revealed,” Angew. Chem. 126, 7874 (2014). doi: 10.1002/anie.201404537
ALS SCIENCE HIGHLIGHT #300