Copper-based catalysts are widely used in chemical industries to convert water and carbon monoxide to hydrogen, carbon dioxide, and methanol. There are theoretical models used to explain this reaction, but a complete understanding of the process has been lacking. However, recent research at the ALS has shed light on the process, giving scientists key data about how copper-based catalysts function at the atomic level. These catalysts are important tools in industrial applications, including partial oxidation of methanol, synthesis of methanol and other derived fuels from CO2, and production of hydrogen. A better understanding of them could pave the way for better catalyst designs.
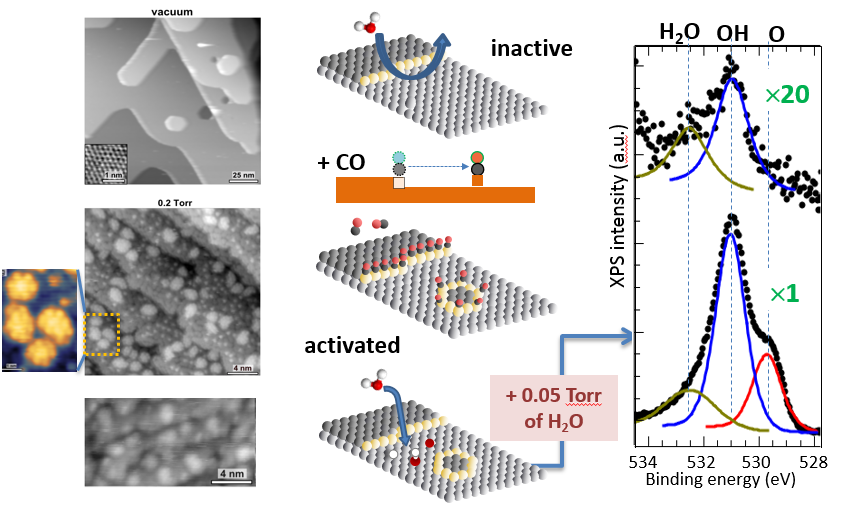
Catalysts are the workhorses of chemical industries. But both in industrial and scientific research, 99 percent of the studies on catalyst surfaces are at the macroscopic scale. Understanding the catalyst surfaces at the atomic level is not an area that’s been fully explored. Prior to this research, there were essentially two theoretical models used to explain copper-based catalyst reactions—either that water dissociates fully to oxygen and hydrogen or that water first couples with carbon monoxide and then dissociates. The second mechanism was predicted to be the more favorable pathway. However at the atomic level, the catalyst surface is never “frozen” as in the theoretical models, but rather changes depending on ambient conditions. “Ambient pressure surface science” was made possible with the developments of the new microscopy and spectroscopy techniques at Berkeley Lab and ALS over the last two decades, and the pioneering work in this field by Berkeley Lab scientist Miquel Salmeron.
Researchers from Berkeley Lab set out to use the capabilities at ALS Beamline 11.0.2 to understand the response of the atomic structure of Cu(111), the most compact and stable surface of copper, to the presence of carbon monoxide (CO) in the gas phase. Scanning tunneling microscopy revealed that the (111) surface of copper becomes unstable when exposed to CO gas, and restructures to form nano-clusters. The new surface is highly active for water dissociation reactions, which changes currently held assumptions about this process. The surface becomes completely unstable at the atomic level, causing it to readily dissociate water, making the first theoretical reaction pathway more favorable.
Scientists already knew that open copper surfaces are active in dissociating water, but the (111) surface, which is the most abundant and most stable face, was completely inactive. With insights gained from this recent ALS research, scientists now know that CO is required to activate the (111) surface. Ambient-pressure scanning tunneling microscopy (AP-STM) provided a view of the atomically resolved surface of copper in the presence of CO. Ambient-pressure x-ray photoelectron spectroscopy (AP-XPS) at Beamline 11.0.2 helped the researchers understand the water dissociation pathway. AP-XPS was developed at Berkeley Lab, and the ALS is currently the only user facility in the world with two beamlines dedicated to this technique.
The (111) faces of cubic metals are very stable, so although many other surfaces can change in the presence of reactive gases, such dramatic changes on a (111) surface have never before been observed. Such a phenomenon is a double effect of the low cohesive energy copper compared to other metals and the gain of energy through carbon monoxide adsorption on the newly formed active sites. This research opens a new paradigm in surface science: Do other soft metals (e.g., gold, silver, zinc) behave the same, and can scientists observe similar phenomena with other reactant gases (e.g., alcohols, carbon dioxide)? Looking for the answers to these questions is the next step in this research process.
Contacts: Miquel Salmeron, Baran Eren, and Danylo Zherebetskyy
Research conducted by: B. Eren, D. Zherebetskyy, L. L. Patera, C.H. Wu, H. Bluhm (Berkeley Lab), L.W. Wang, G.A. Somorjai, Miquel Salmeron; C. Africh (Laboratorio STASC).
Research funding: U.S. Department of Energy (DOE), Office of Basic Energy Sciences (BES). Operation of the ALS is supported by DOE BES.
Publication about this research: B. Eren, D. Zherebetskyy, L. L. Patera, C.H. Wu, H. Bluhm, C. Africh, L.W. Wang, G.A. Somorjai, and Miquel Salmeron, “Activation of Cu(111) surface by decomposition into nanoclusters driven by CO adsorption,” Science 351, 475 (2016).
ALS SCIENCE HIGHLIGHT #330