SCIENTIFIC ACHIEVEMENT
Using a combination of probes, including nanoscale spectroscopy at the Advanced Light Source (ALS), researchers found that twisting 2D layers at atypically large angles opens up potentially useful energy gaps in the material’s band structure.
SIGNIFICANCE AND IMPACT
The results suggest a new way to tune materials for optoelectronic applications and provide a platform for exploring novel “moiré” phenomena beyond those observed at small twist angles.
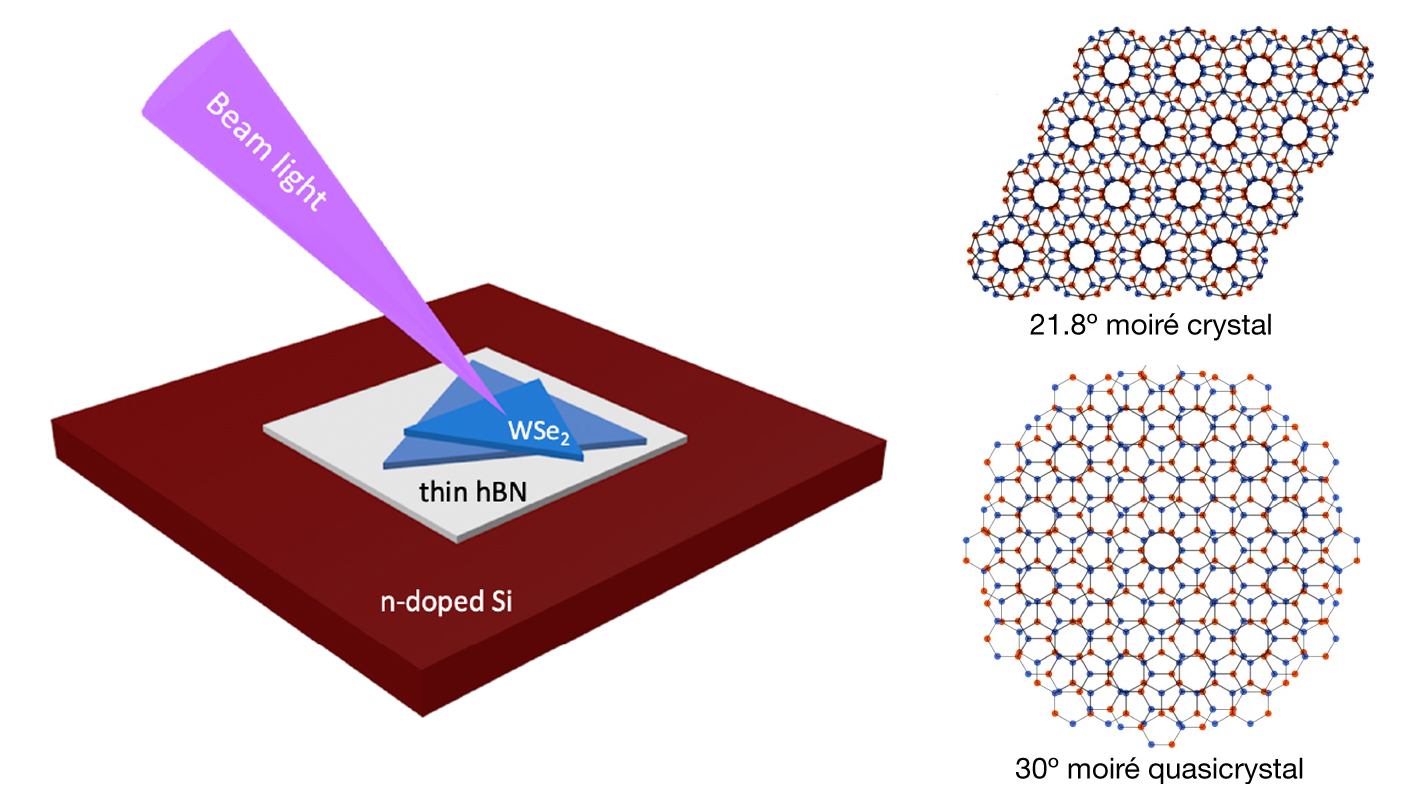
A new frontier in moiré physics
The discovery of emergent electronic properties in stacks of two-dimensional materials has led to a surge in efforts to engineer layered heterostructures with new functional properties. One fascinating twist in the story was the revelation that, by slightly rotating two layers with respect to each other, a moiré-effect “superlattice” can be generated. This extra level of organization induces modifications to the material’s electronic properties that could prove to be useful—in optoelectronic applications, for example. Thus far, most superlattices investigated have been in bilayers with small twist angles that result in a crystalline moiré pattern, characterized by repeating unit cells.
In this work, a form of 2D material was created by twisting the layers at a larger angle, leading to an aperiodic “quasicrystalline” moiré lattice with a more complex structure and whose electronic properties are hard to predict. The material was characterized using advanced spectroscopic techniques that demonstrated the presence of rich band-structure features—valleys and minigaps—that present opportunities for tuning electronic properties. The results establish bilayers with large twist angles as a viable platform for exploring “moiré physics.”
The search for valleys and minigaps
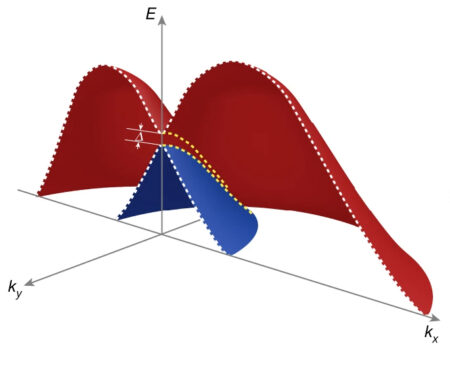
Here, researchers produced moiré quasicrystals from tungsten diselenide (WSe2) bilayers twisted at a 30° angle. They used scanning tunneling spectroscopy (STS) to probe the density of electronic states at several points on the quasicrystal surface. At each location, peaks in the density of states were assigned to specific maxima and minima (both known as “valleys”) in the band structure.
By analyzing this data, the researchers could infer the positions and sizes of minigaps—small splittings in band crossings that open up due to the interactions of valleys in the top and bottom layers. This was very exciting because one could conceivably tune the minigaps (e.g., by doping) to affect the absorption or emission of light. However, before the minigaps could be conclusively attributed to band-structure splitting (as opposed to, say, disorder or defects in the material), more information was needed.
NanoARPES experiments at the ALS
To obtain definitive proof that the minigaps are features of the material’s intrinsic electronic structure, nanoscale-resolution ARPES experiments were performed at ALS Beamline 7.0.2. Complementary to the STS data, where measurements reveal the presence of valleys and minigaps in the material averaged over real space, ARPES directly measures not just the energy but also the momentum of electrons, and thus is capable of revealing the band structure in momentum space. The nanoARPES experiments were extremely challenging, but were made possible by the high quality of the beamline and the expertise of the staff at the ALS.
With the nanoARPES data, the researchers were able to demonstrate a clear correspondence between STS peaks and specific valleys in the band structure (the K-valley and the Γ-valley), and that the places where the K-valleys intersect is where the STS data revealed minigaps. When put together, the consistency of the results eliminates other possible explanations for the STS data. Overall, the results show that large-twist-angle moiré quasicrystals like WSe2 exhibit a rich interplay of valley and spin degrees of freedom that can provide new tuning knobs for optical and transport properties.
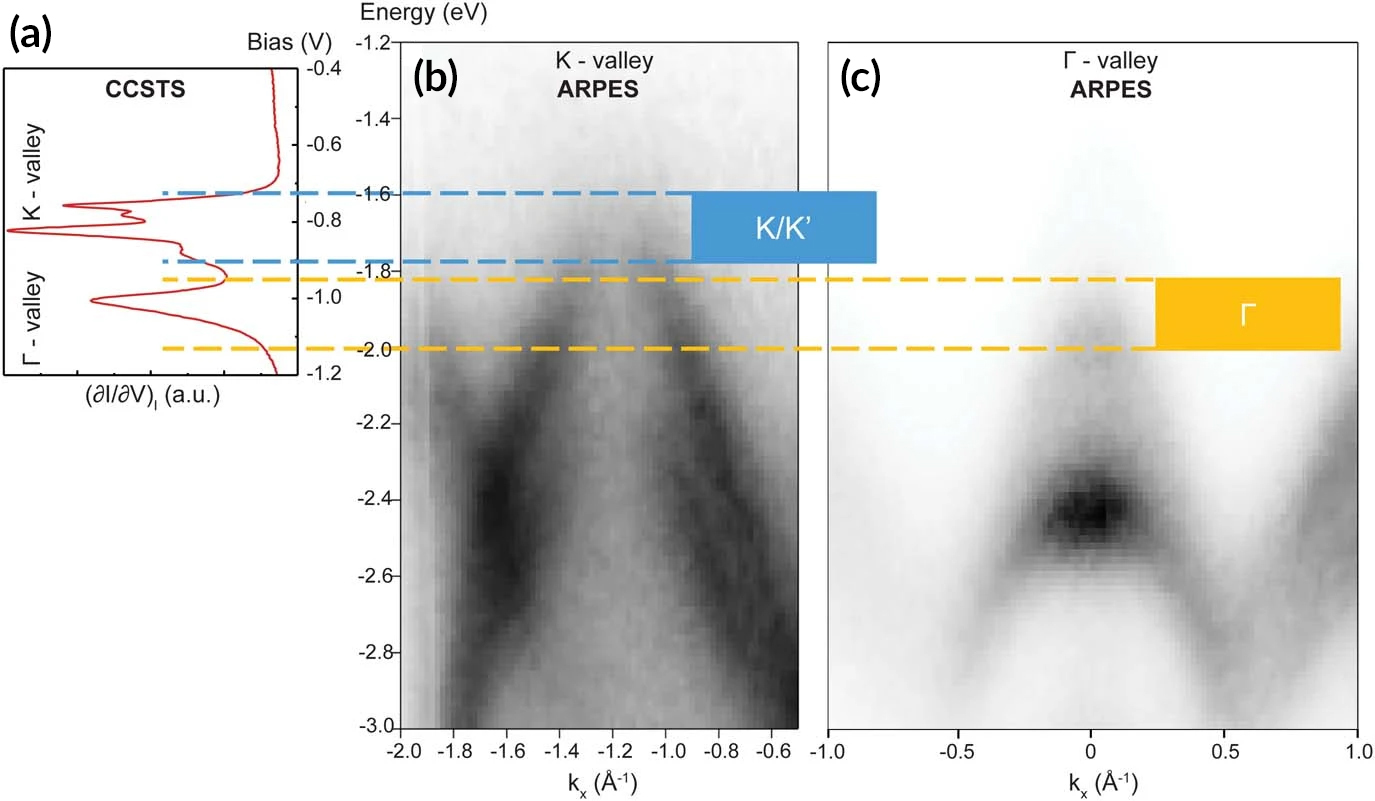
Contact: Eli Rotenberg
Researchers: Y. Li, F. Zhang, V.-A. Ha, Q. Gao, Z. Liu, X. Liu, H. Kim, B. Kousa, X. Li, E. Khalaf, F. Giustino, and C.K. Shih (University of Texas at Austin); Y.-C. Lin (Pennsylvania State University and National Yang Ming Chiao Tung University, Taiwan); C. Dong and J.A. Robinson (Pennsylvania State University); S.H. Ryu, C. Jozwiak, A. Bostwick, and E. Rotenberg (ALS); and K. Watanabe and T. Taniguchi (National Institute for Materials Science, Japan).
Funding: National Science Foundation; US Air Force; Welch Foundation; US Department of Energy, Office of Science, Basic Energy Sciences program (DOE BES) and National Quantum Information Science Research Centers; National Yang Ming Chiao Tung University; Ministry of Education of Taiwan; and Japan Society for the Promotion of Science. Operation of the ALS is supported by DOE BES.
Publication: Y. Li, F. Zhang, V.-A. Ha, Y.-C. Lin, C. Dong, Q. Gao, Z. Liu, X. Liu, S.H. Ryu, H. Kim, C. Jozwiak, A. Bostwick, K. Watanabe, T. Taniguchi, B. Kousa, X. Li, E. Rotenberg, E. Khalaf, J.A. Robinson, F. Giustino, and C.K. Shih, “Tuning commensurability in twisted van der Waals bilayers,” Nature 625, 494 (2024), doi:10.1038/s41586-023-06904-w.
ALS SCIENCE HIGHLIGHT #501