SCIENTIFIC ACHIEVEMENT
Using the Advanced Light Source (ALS), researchers found that, in crystals with structural chirality (left- or right-handedness), tuning the electronic behavior reveals hidden chiral phases and singularities.
SIGNIFICANCE AND IMPACT
The results provide a new way to predict, test, and manipulate novel materials that exhibit desirable properties for next-generation electronic and spintronic devices.
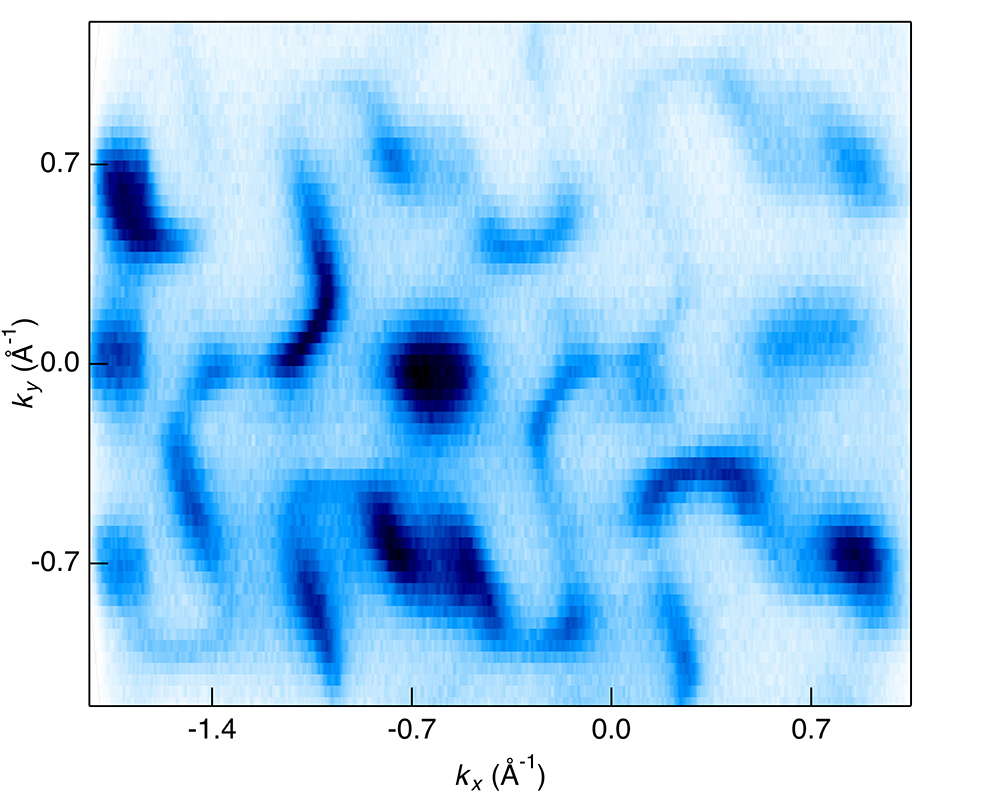
A Look in the Mirror Reveals New Physics
Chiral objects have two nearly identical versions: right- and left-handed. Just like your hands or your feet, chiral objects are mirror images that cannot be superimposed on each other through any rotational transformation. Chirality is a key principle in cutting-edge materials research because it breaks crystal symmetries, a condition that often leads to new physical phenomena with relevance to next-generation electronic and spintronic devices.
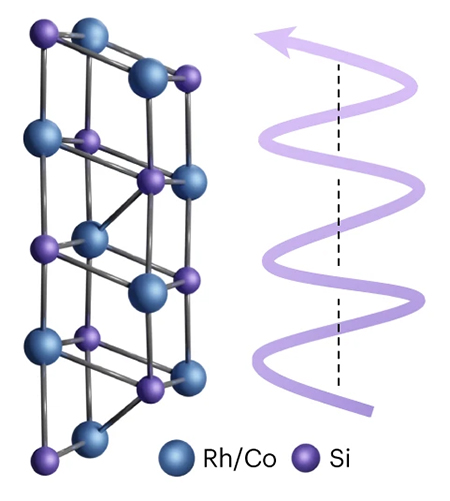
One example of the consequences of chirality in materials involves the peculiar helical trajectories of electrons in crystals with chiral atomic structures. In earlier work, physicists at Princeton University predicted and confirmed a link between this type of crystal-lattice chirality and the chiral momentum distribution of topologically protected electrons at the crystal surface. These chiral electronic behaviors manifested as looping, helicoid “Fermi arcs” in spectroscopic data obtained at the ALS.
Now, in two recently published studies, the Princeton group has extended their study of topological chiral crystals by tuning the crystals’ electronic structure using chemical doping. This enabled them to probe multiple intertwined topological phases of the surface electrons as well as saddle-shaped features (“van Hove singularities”) associated with the emergence of exotic material properties.
Tuning into the multigaps
The researchers performed angle-resolved photoemission spectroscopy (ARPES) on two chiral crystals, RhSi and CoSi. Ultraviolet light from ALS Beamline 4.0.3 was used to probe the surface electronic states, while soft x-rays (at the Swiss Light Source and SPring-8 in Japan) probed the bulk states. Because some of the bulk bands of interest were at a level inaccessible to photoemission techniques (i.e., above the Fermi level), the researchers shifted the band structure downward by doping with small amounts of Ni.
In RhSi, this allowed visualization of the intersection of three bulk energy bands that defined two gap regions between them. The researchers systematically explored these bulk multigaps and aligned spectral data from the bulk with data from the surface. The correspondences thus revealed between the bulk bands and the surface Fermi arcs (“bulk-boundary correspondence”) provide strong evidence for the topological nature of the chiral surface states.
Emergence of van Hove singularities
Based on their detailed studies, the researchers were also able to predict that the topological properties of these chiral crystals can drive surface electrons to become unstable. Saddle-shaped van Hove singularities in the ARPES data are a signature of such instabilities. In fact, the researchers found two types of van Hove singularity: type I (occurring between helicoid arcs) and type II (occurring within a single helicoid arc).
As unstable surface electrons interact with each other to reduce their energy, exotic new states of matter can emerge—collective excitations or correlated electron states, for example—with impacts on optical characteristics, magnetic order, charge-carrier mobility, and other useful properties.
Ultimately, these discoveries offer scientists a predictive tool for identifying materials that host novel topological effects. Moreover, the ability to tune a system to the van Hove singularity offers an exciting testing ground for the study and manipulation of correlation effects in promising topological materials.
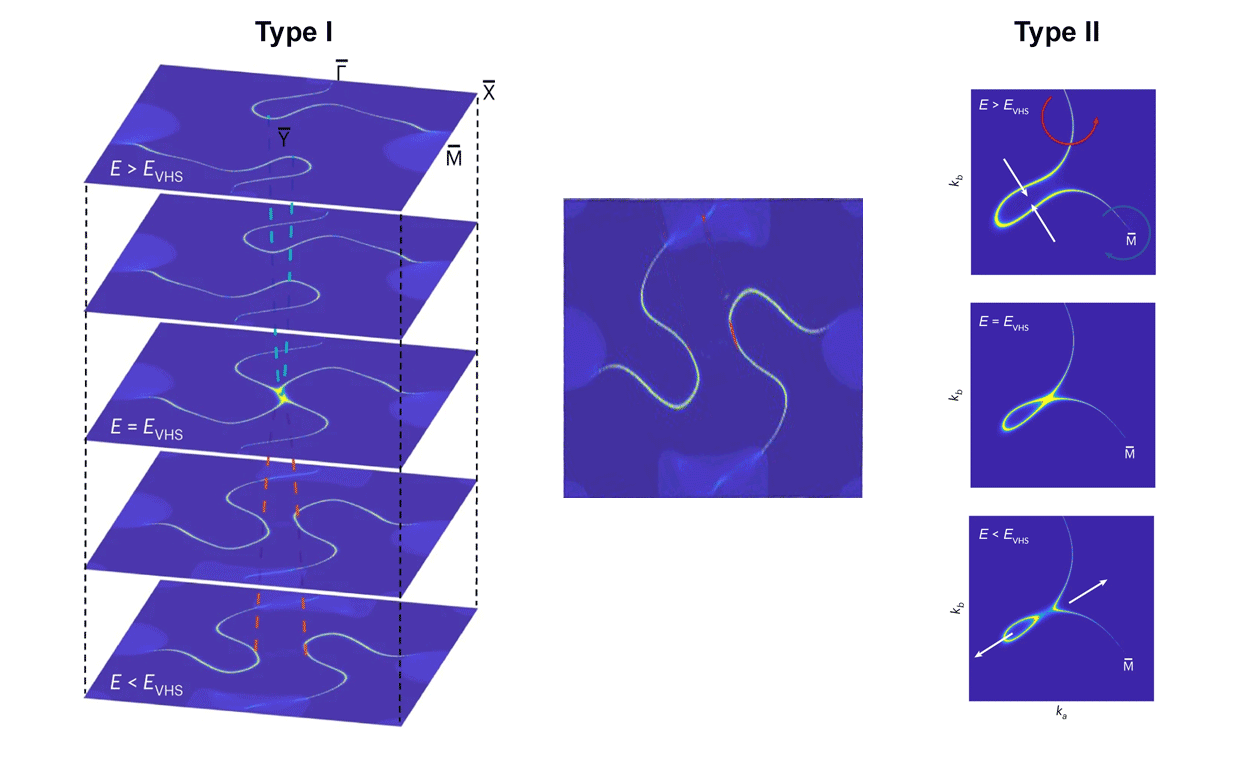
Contact: Tyler Cochran
Researchers: T.A. Cochran, I. Belopolski, D.S. Sanchez, Z.-J. Cheng, X.P. Yang, D. Multer, and J.-X. Yin (Princeton University); K. Manna (Max Planck Institute for Chemical Physics of Solids, Germany, and Indian Institute of Technology, India); M. Yahyavi (National Cheng Kung University, Taiwan, and Nanyang Technological University, Singapore); Y. Liu and X. Xu (Peking University); H. Borrmann and C. Shekhar (Max Planck Institute for Chemical Physics of Solids, Germany); A. Chikina and V.N. Strocov (Swiss Light Source); J.A. Krieger (Swiss Light Source and Paul Scherrer Institute, Switzerland); J. Sánchez-Barriga (BESSY II and IMDEA Nanociencia, Spain); P. Le Fèvre and F. Bertran (SOLEIL Synchrotron); J.D. Denlinger (ALS); T.-R. Chang (National Cheng Kung University, Taiwan); S. Jia, G. Chang, and T. Hou (Nanyang Technological University, Singapore); W. Xie (Michigan State University); C. Felser (Max Planck Institute for Chemical Physics of Solids, Germany); H. Lin (Academia Sinica, Taiwan); and M.Z. Hasan (Princeton University and Berkeley Lab).
Funding: US Department of Energy, Office of Science, Basic Energy Sciences program (DOE BES); National Science Foundation; Helmholtz Association (Germany); European Research Council; Swiss National Science Foundation; National Research Foundation (Singapore); Nanyang Technological University (Singapore); Department of Atomic Energy (India); and Max Planck Society (Germany). Operation of the ALS is supported by DOE BES.
Publication: T.A. Cochran, I. Belopolski, K. Manna, M. Yahyavi, Y. Liu, D.S. Sanchez, Z.-J. Cheng, X.P. Yang, D. Multer, J.-X. Yin, H. Borrmann, A.Chikina, J.A. Krieger, J. Sánchez-Barriga, P. Le Fèvre, F. Bertran, V.N. Strocov, J.D. Denlinger, T.-R. Chang, S. Jia, C. Felser, H. Lin, G. Chang, and M.Z. Hasan, “Visualizing Higher-Fold Topology in Chiral Crystals,” Phys. Rev. Lett. 130, 066402 (2023), doi:10.1103/PhysRevLett.130.066402; and D.S. Sanchez, T.A. Cochran, I. Belopolski, Z.-J. Cheng, X.P. Yang, Y. Liu, T. Hou, X. Xu, K. Manna, C. Shekhar, J.-X. Yin, H. Borrmann, A. Chikina, J.D. Denlinger, V.N. Strocov, W. Xie, C. Felser, S. Jia, G. Chang, and M.Z. Hasan, “Tunable topologically driven Fermi arc van Hove singularities,” Nat. Phys. 19, 682 (2023), doi:10.1038/s41567-022-01892-6.
ALS SCIENCE HIGHLIGHT #485