Radiation therapy is currently the standard of care for more than half of all cancer patients. Despite technological advances that have improved its power and precision, conventional radiotherapy still has its limitations, particularly for radiation-resistant tumors. Cranking up the delivery dose, which is often required to completely eliminate cancerous tissue, can induce serious side effects in healthy surrounding tissue.
Though it may seem counterintuitive, delivering ultrafast, high-intensity doses of radiation to tumors can actually reduce toxicity to surrounding healthy cells, while still directing a potent anti-cancer effect toward the target. Dubbed the FLASH radiotherapy effect, scientists have yet to confirm how or why it works.
“It’s really surprising that higher dose rates can actually protect tissues while still destroying tumor tissue,” said Corie Ralston, a staff scientist in Berkeley Lab’s Molecular Biophysics and Integrated Bioimaging Division and director of the biological nanostructures facility at the Molecular Foundry. “It’s a giant puzzle.”
To clarify the underlying mechanisms of the FLASH effect, researchers directly compared conventional and FLASH techniques, using a short peptide in water as an experimental model. For the low-dose-rate, conventional radiation condition, they irradiated peptide samples on a self-contained x-ray system; for the ultrafast, FLASH radiation condition, they irradiated samples at Advanced Light Source (ALS) Beamline 3.3.1. X-ray footprinting mass spectrometry (XFMS) uses x-rays to precisely probe the oxidation patterns and reactivity of proteins in water. Fewer radiation-induced changes occurred in peptides at ultrafast FLASH rates, consistent with previous reports.
One theory for how FLASH radiotherapy allows tissues to tolerate higher doses is that the rapid delivery of radiation induces oxygen depletion, providing a protective effect for normal tissues, but not cancer cells. To investigate this, the researchers ran the experiment under both oxygen-rich and oxygen-poor conditions, and observed lower oxidation rates in the oxygen-starved peptides. Although this does not confirm the precise mechanism of the FLASH effect, it does point to oxygen as a critical player.
The researchers hope to build on their work in simple peptides to study the FLASH effect first in full proteins, then cell lines, and eventually both healthy and cancerous tissues.
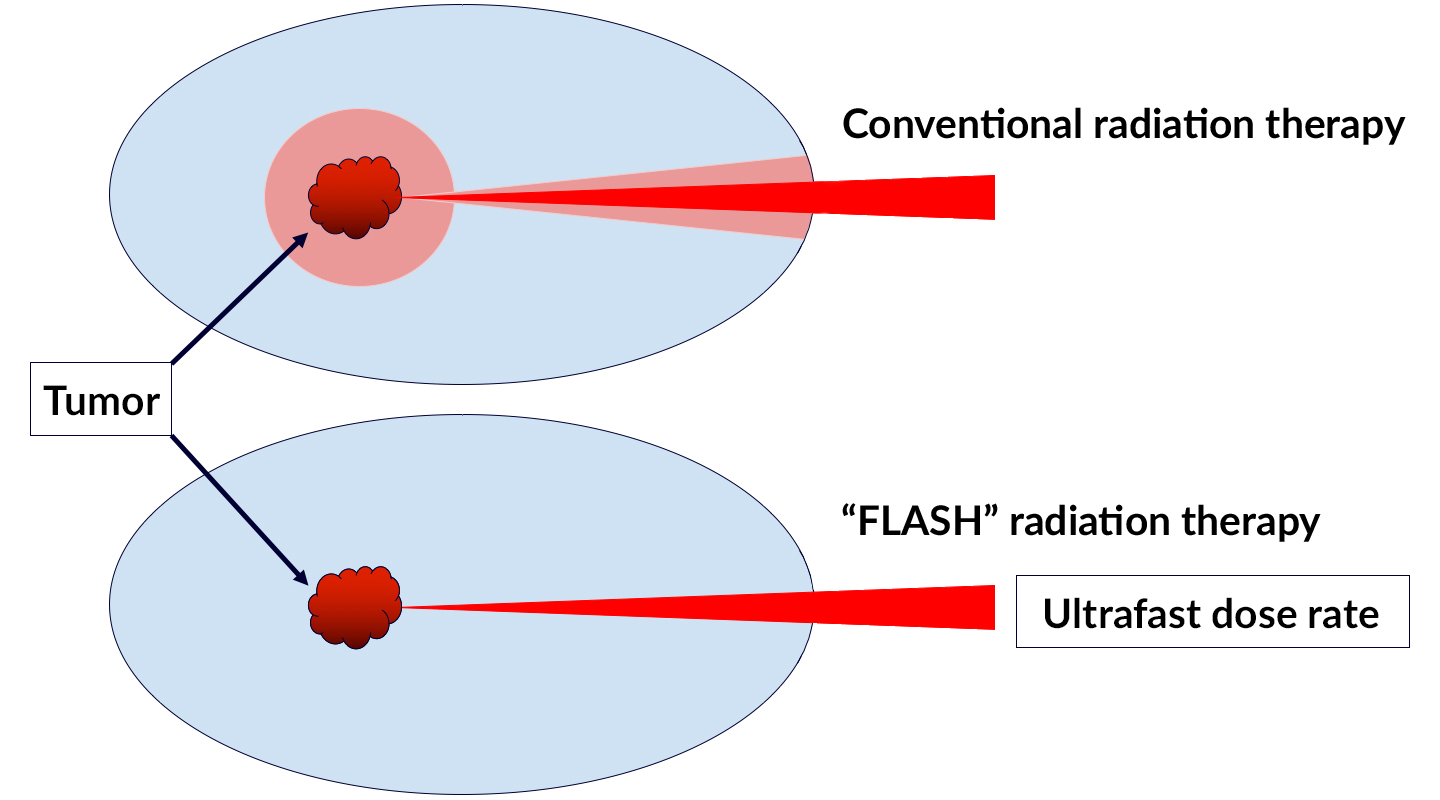
S. Gupta, J.L. Inman, J. De Chant, L. Obst-Huebl, K. Nakamura, S.M. Costello, S. Marqusee, J.-H. Mao, L. Kunz, R. Paisley, M.-C. Vozenin, A.M. Snijders, and C.Y. Ralston, “A Novel Platform for Evaluating Dose Rate Effects on Oxidative Damage to Peptides: Toward a High-Throughput Method to Characterize the Mechanisms Underlying the FLASH Effect,” Rad. Res. 200, 523 (2023), doi:10.1667/RADE-23-00131.1.
Adapted from Berkeley Lab’s Biosciences Area highlight, “Towards a New Framework for Radiation Cancer Treatment.”