SCIENTIFIC ACHIEVEMENT
Soft x-ray studies of hematite electrodes—potentially key components in producing fuel from sunlight—revealed the material’s electronic band positions under realistic operating conditions.
SIGNIFICANCE AND IMPACT
By clarifying the mechanisms limiting hematite’s photoelectrochemical (PEC) performance, this work brings us a step closer to directly converting solar energy into easily storable chemical fuel.
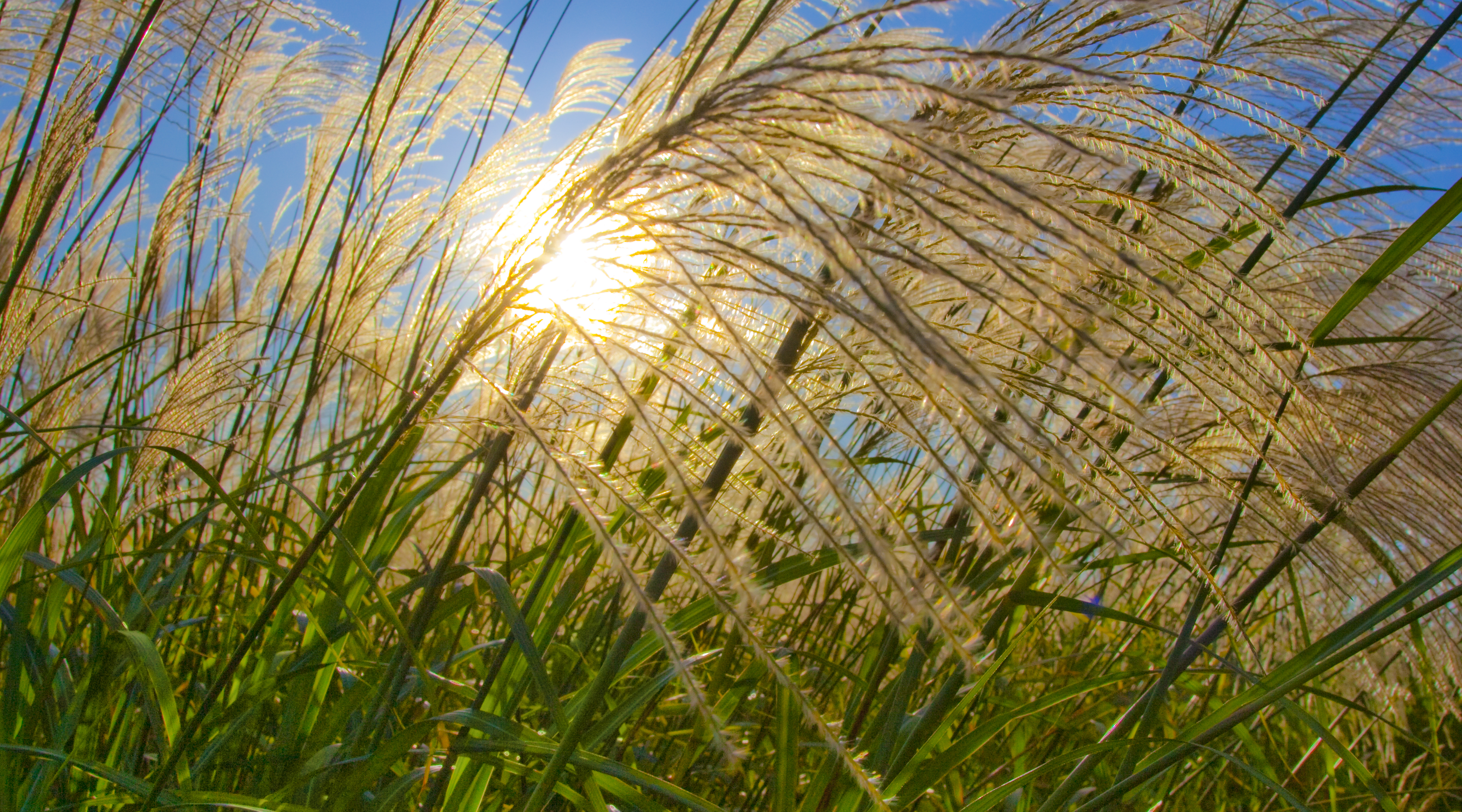
Water splitting—key to photosynthesis
In photosynthesis, plants use sunlight to split water into oxygen and hydrogen. The oxygen is released into the atmosphere, and the hydrogen is used to produce molecules—such as carbohydrates and sugars—that store energy in chemical bonds. Such compounds constitute the original feedstocks for subsequent forms of fuel consumed by society.
Photoelectrochemical (PEC) water splitting is a form of “artificial” photosynthesis that uses semiconductor material, rather than organic plant material, to facilitate water splitting. Electrodes made of semiconductor material are immersed in an electrolyte, with sunlight driving the water-splitting process. The performance of such PEC devices is largely determined at the interface between the photoanode (the electrode at which light gets absorbed) and the electrolyte.
Probing the hematite/electrolyte interface
A widely studied photoanode material is hematite (α-Fe2O3), a cheap, earth-abundant semiconductor with a favorable band gap and high chemical stability under the harsh conditions of many PEC reactions. However, it’s still not efficient enough to be widely used in these devices. To gain insight into why, the researchers studied thin (30-nm) films of hematite, a thickness optimized for maximum PEC performance. They studied both doped and undoped hematite to learn from their different behaviors about the root causes for limited PEC performance.
APXPS experiments with “tender” x-rays
Past experiments at the ALS have shown that ambient-pressure x-ray photoelectron spectroscopy (APXPS) can be used to study the heterogeneous interfaces of aqueous solutions. It provides an interface-specific, noncontact probe based on measuring the kinetic energy of photoelectrons originating from the interface. It’s also an excellent tool for measuring changes in interfacial electrical potentials. This is because electrons, being charged particles, change their kinetic energy (i.e., “speed”) when the electrical potential at the interface is changing. With these capabilities, the researchers were able to directly measure shifts in valence and conduction band positions at the hematite/electrolyte interface of an operating PEC cell as a function of solar illumination, applied potential, and doping.
For this work, the researchers took a liquid cell normally mounted on Beamline 11.0.2 and moved it to Beamline 6.0.1, a source of “tender” x-rays (about 3.5 keV). Photoelectrons generated by the tender x-rays have kinetic energies (about 2 keV and higher) capable of penetrating the thin electrolyte film in contact with the hematite.
Missing “in-gap” states
Surprisingly, the data showed that both doped and undoped hematite samples exhibited so-called “ideal semiconductor” behavior, appearing to be unaffected by an expected interfacial effect (“Fermi-edge pinning”) that would allow electronic states to populate the band gap, where they are normally forbidden. A commonly accepted scenario assumes that in-gap states act as trap centers for holes, increasing the probability for hole recombination with electrons, thus degrading performance.
The results contradict previous reports (based on less-direct methods) that in-gap states are responsible for the decreased PEC performance of hematite. Instead, the results imply that the poor PEC performance of hematite is due to other reasons, such as the small charge-carrier concentration for undoped hematite and tunneling-mediated recombination of electrons and holes in the case of doped hematite.
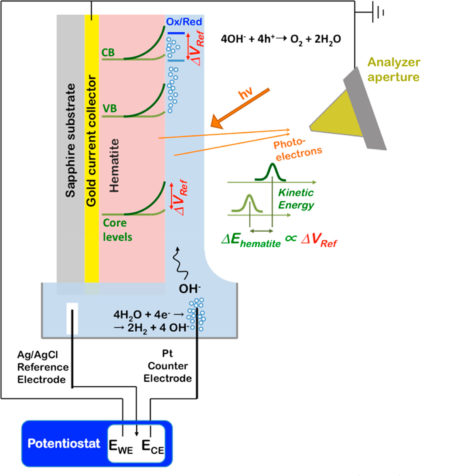
Contact: Hendrik Bluhm
Researchers: A. Shavorskiy, O. Karslıoğlu, M. Hartl, I. Zegkinoglou, L. Trotochaud, S. Nemsak, and P.N. Ross (Berkeley Lab); X. Ye, A.D. Poletayev, and W. Chueh (Stanford University); C.M. Schneider (Forschungszentrum Jülich, Germany); E. Crumlin, S. Axnanda, and Z. Liu (ALS); and H. Bluhm (Berkeley Lab and ALS).
Funding: Global Climate and Energy Project at Stanford University, Stanford University, and U.S. Department of Energy, Office of Science, Basic Energy Sciences Program (DOE BES). Operation of the ALS is supported by DOE BES.
Publication: A. Shavorskiy, X. Ye, O. Karslıoğlu, A.D. Poletayev, M. Hartl, I. Zegkinoglou, L. Trotochaud, S. Nemsak, C.M. Schneider, E. Crumlin, S. Axnanda, Z. Liu, P.N. Ross, W. Chueh, and H. Bluhm, “Direct mapping of band positions in doped and undoped hematite during photoelectrochemical reactions,” J. Phys. Chem. Lett. 8, 5579 (2017), doi:10.1021/acs.jpclett.7b02548.
ALS SCIENCE HIGHLIGHT #366