Scientists are seeking ways to reduce levels of carbon dioxide (CO2) in the atmosphere by improving the processes that convert CO2 gas into other compounds such as ethanol (a liquid fuel). Copper is currently considered the best catalyst for this process; however, it is not very efficient. Ambient-pressure x-ray photoelectron spectroscopy (APXPS) at the ALS, coupled with theoretical work by a team at the California Institute of Technology, revealed how oxygen atoms embedded very near the surface of a copper sample dramatically boost the early stages of the CO2 catalytic reaction. The information could prove useful in designing new types of materials to further enhance reactions and make them more efficient in converting CO2 into other products.
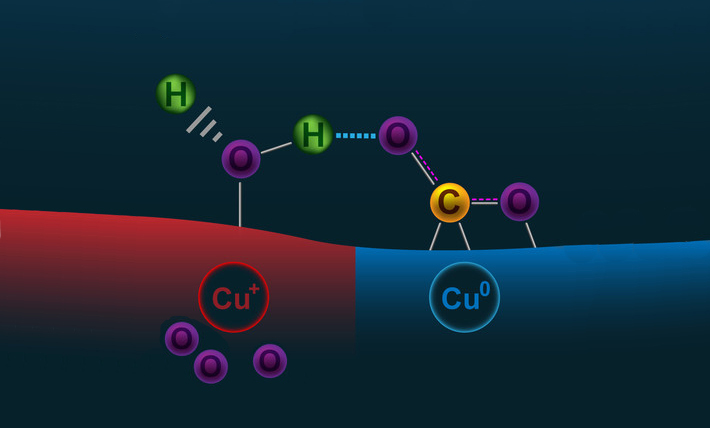
Large concentrations of CO2 are harmful to human health and the environment, so researchers have been pursuing ways to remove it from the atmosphere and safely store it or chemically convert it into more beneficial forms. To convert CO2 into valuable hydrocarbons, a source of protons is needed, with water (H2O) being the favorite choice. However, CO2 is highly inert, and a catalyst is needed to initiate CO2 conversion reactions. Copper is the most promising pure-metal catalyst, but it is not very efficient or scalable, and its reaction pathways generally don’t lead to the desired final products. Despite numerous experimental and theoretical studies, the role played by the copper surface structure in initiating chemical reactions is still unclear, and scientists lack a comprehensive understanding of how CO2 and H2O molecules adsorb on the copper surface and interact.
At ALS Beamline 9.3.2, researchers performed APXPS experiments on thin foils of copper exposed to pure CO2 gas as well as mixtures of CO2 and H2O at room temperature. In some of the experiments, the sample was heated slightly in oxygen to increase the concentration of oxygen embedded in the foil. The APXPS data then revealed the various species of carbon and oxygen at the copper surface in the presence of water and different amounts of subsurface oxide. The probe depth (determined by the mean free path of the escaping photoelectrons) was about 1.2 nm from the topmost layer. At Caltech, members of the research team worked to develop and refine a quantum mechanical theory that unraveled the x-ray observations and explained the behavior of the molecules in the reaction.
The results showed that when there is little to no subsurface oxygen, CO2 molecules (in their usual linear O=C=O arrangement) hover above the copper surface in a weakly bound, physisorbed state (adsorption involving van der Waals forces). A modest amount of subsurface oxygen causes some of the copper near the surface to become positively charged. This has the effect of stabilizing any adsorbed H2O molecules, which can then interact with nearby CO2 molecules to facilitate chemisorbtion (adsorption involving chemical bonds), bending them through an angle of about 120°. This chemisorption is a key step in the transformation of CO2 and H2O into formate (HCOO–) and formic acid (HCOOH), which are basic building blocks for more complex hydrocarbons such as ethanol. Too much subsurface oxygen, however, decreases the stability of the CO2 and lowers the catalytic activity. The calculations indicated that an optimum amount of subsurface oxygen is (at most) one oxygen per every four copper atoms, or Cu(111)Ox=0.25.
The researchers expect that it should be possible to mix and match different catalysts (e.g., other copper surfaces, copper alloys, or other metals) with subsurface atoms other than oxygen (e.g., sulfur or chlorine). The insights gained in this work have thus provided a foundation for the rational development of improved CO2 catalysis as well as unique active electrocatalysts in general.
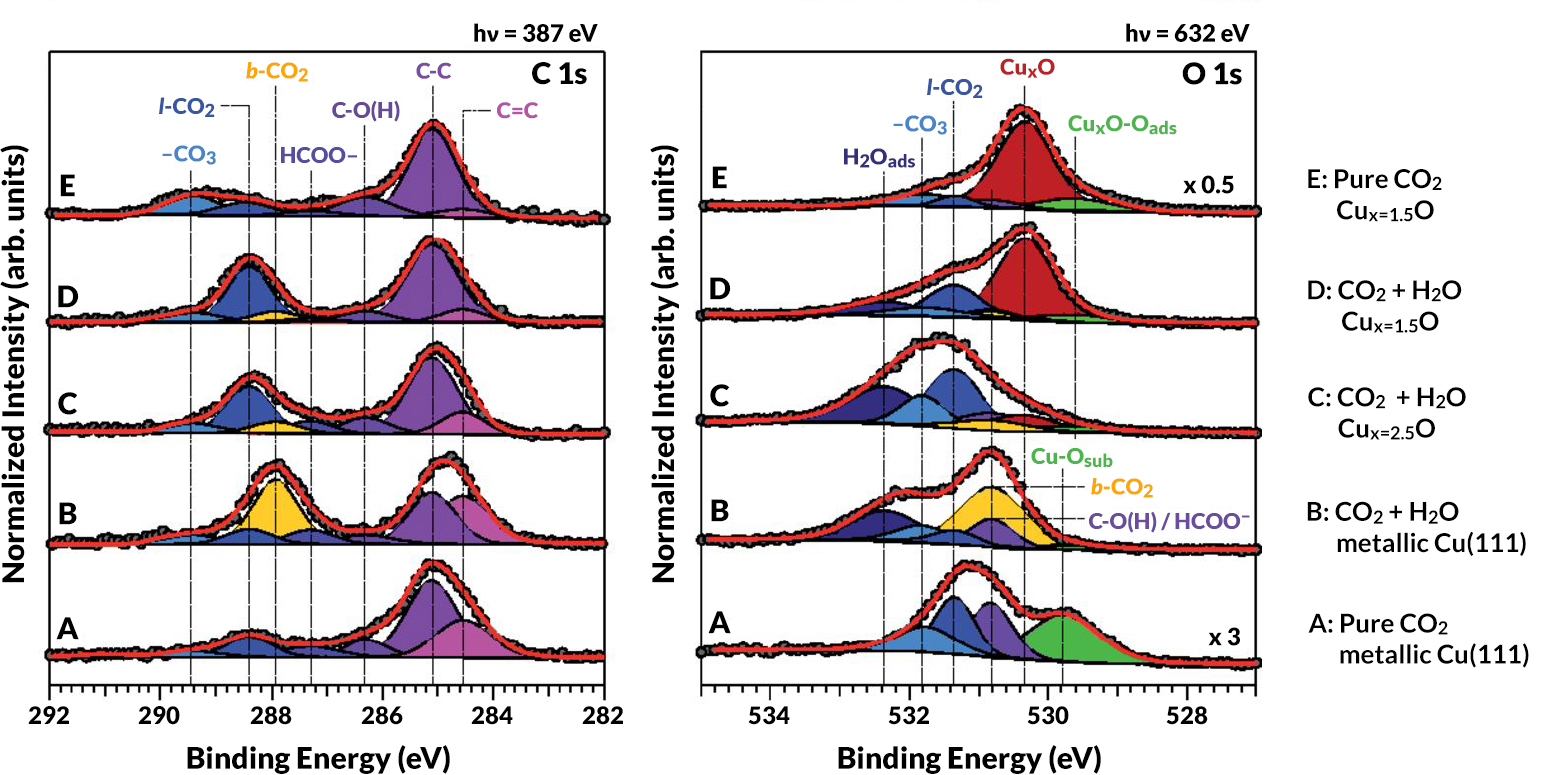
Contact: Ethan Crumlin
Research conducted by: M. Favaro and J. Yano (Berkeley Lab); H. Xiao, T. Cheng, and W.A. Goddard, III (California Institute of Technology); and E.J. Crumlin (ALS).
Research funding: U.S. Department of Energy, Office of Science, Basic Energy Sciences Program (DOE BES). Operation of the ALS is supported by DOE BES.
Publication about this research: M. Favaro, H. Xiao, T. Cheng, W.A. Goddard, III, J. Yano, and E.J. Crumlin, “Subsurface oxide plays a critical role in CO2 activation by Cu(111) surfaces to form chemisorbed CO2, the first step in reduction of CO2,” PNAS 114, 6706 (2017). doi: 10.1073/pnas.1701405114
Adapted from the Berkeley Lab news release, “Researchers Find a Surprise Just Beneath the Surface in Carbon Dioxide Experiment” and the Caltech news release, “Researchers Find a Surprise Just Beneath the Surface in Carbon Dioxide Experiment.”
ALS SCIENCE HIGHLIGHT #359