SCIENTIFIC ACHIEVEMENT
Using high-pressure radial x-ray diffraction at the Advanced Light Source (ALS), researchers found that in the superhard material, rhenium diboride, smaller grain size leads to greater yield strength (i.e., the amount of stress tolerated before permanent deformation).
SIGNIFICANCE AND IMPACT
Because transition-metal borides are extremely hard, metallic, and can be synthesized at ambient pressure, they have exciting potential for use in next-generation cutting tools.
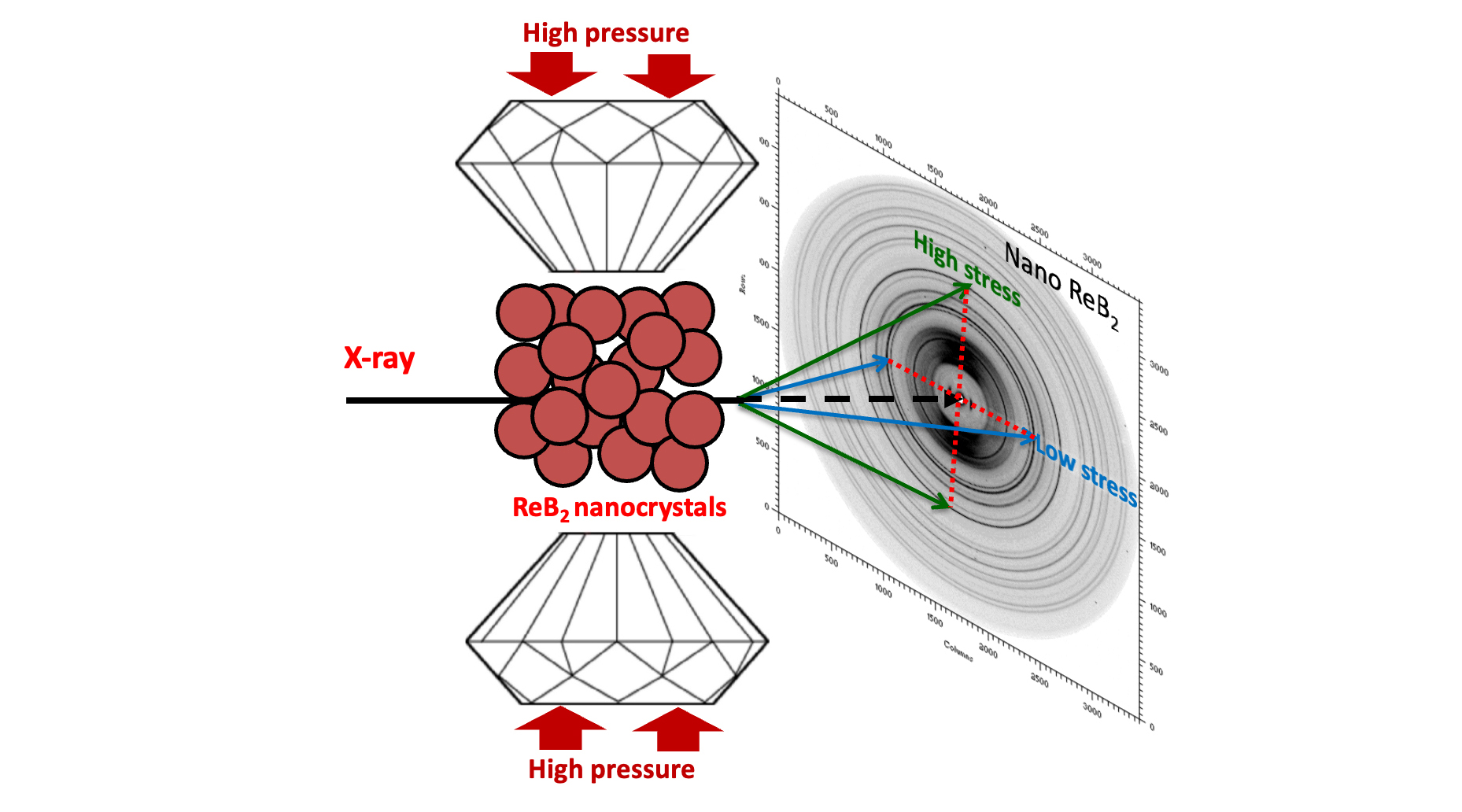
The cutting edge
As we get better at making robust, durable materials that resist wear, corrosion, and extreme temperatures—think aerospace, automotive, and industrial machining applications—we also need to step up the quality of the tools we use to cut, form, and polish these hardened materials. Researchers have found that transition-metal borides are promising in this regard because of their low costs and advantageous mechanical properties. Metal borides combine highly incompressible transition metals (e.g., tungsten, rhenium, and osmium) with boron, which readily forms strong covalent bonds—a key characteristic of the prototypical superhard material, diamond. In this work, researchers used radial x-ray diffraction at high pressures to gain insight into how grain size affects the nanoscale deformation mechanics of the superhard material, rhenium diboride (ReB2).
Inspired by diamonds
Diamond is a well-known superhard material. But diamond production requires both high temperatures and high pressures, both of which naturally occur deep underground. To replicate the hardness of diamond under ambient conditions, researchers have looked for materials that incorporate two diamond-like characteristics: densely packed electrons and a highly covalent bonding network.
Electron-dense, incompressible elements can be found toward the bottom of the periodic table, but those elements are also metals, characterized by malleability and ductility. A promising strategy is to combine the metals with boron, which readily forms strong covalent bonds. The first example of a superhard metal boride following this design principle was ReB2, consisting of alternating layers of rhenium and boron. While size-induced hardening has been previously studied in softer inorganic materials, size effects in hard materials have been relatively unexplored.
High-pressure radial x-ray diffraction
In this work, the researchers synthesized ReB2 powder samples with grain sizes of 20, 50, and 60 nm. They then used high-pressure radial x-ray diffraction at ALS Beamline 12.2.2—a dedicated high-pressure beamline—to explore the effect of grain size on yield strength, which is directly related to hardness. The technique uses a diamond-anvil cell to compress the sample (up to 50 GPa), with the x-ray beam perpendicular to the compression axis. This creates differential compressive stresses along the various crystal planes and allows measurement of changes in the crystal lattice parameters. From measurements of the lattice parameters, the researchers calculated differential stress, which they then plotted vs pressure for three different lattice planes: the (002) plane, parallel to the rhenium and boron layers, and (101) and (110), which cut through the layers.
Nanoscale deformation mechanisms
In the differential stress plots, a plateau indicates slippage of lattice planes (stress remains constant even as pressure increases). In contrast, an increasing trend indicates resistance to slipping (higher pressures generate higher stress). The lowest plateau occurred in the (002) plane, and the smallest grains exhibited the most slip resistance. This latter behavior likely arises from an increased concentration of grain boundaries, which act to halt the spread of dislocations. In addition to lattice parameters and stress states, the diffraction experiments provided data for texture analysis, which reinforced the study’s main conclusions. Overall, the work demonstrates that control over grain size and boundary effects in superhard materials can be used to produce new materials with even more impressive mechanical properties.
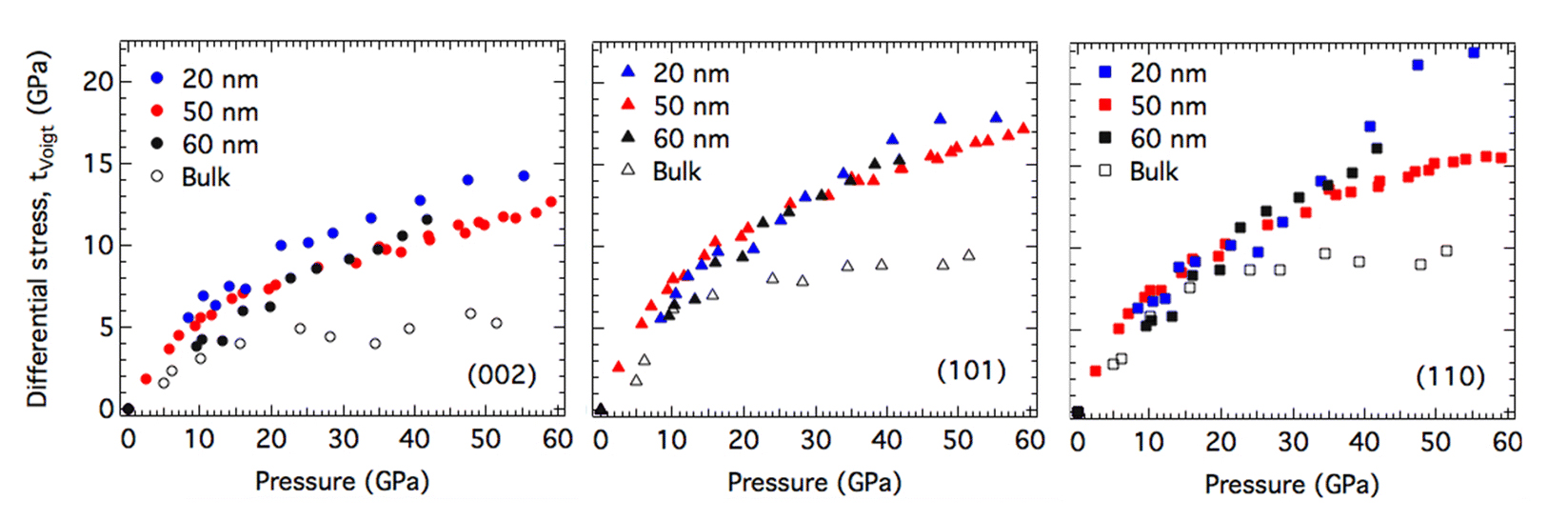
Contact: Sarah Tolbert
Researchers: S. Hu, S.G. Hamilton, C.L. Turner, D.D. Robertson, A. Kavner, R.B. Kaner, and S.H. Tolbert (Univ. of California, Los Angeles), and J. Yan (ALS).
Funding: National Science Foundation, Consortium for Materials Properties Research in Earth Sciences. Operation of the ALS is supported by the US Department of Energy, Office of Science, Basic Energy Sciences program.
Publication: S. Hu, S.G. Hamilton, C.L. Turner, D.D. Robertson, J. Yan, A. Kavner, R.B. Kaner, and S.H. Tolbert, “High-pressure studies of size dependent yield strength in rhenium diboride nanocrystals,” Nanoscale Horiz. 9, 646 (2024), doi:10.1039/D3NH00489A.
ALS SCIENCE HIGHLIGHT #507