SCIENTIFIC ACHIEVEMENT
Researchers used the Advanced Light Source (ALS) to help demonstrate that coupled electronic and magnetic properties in a material can be repeatably tuned using laser light.
SIGNIFICANCE AND IMPACT
The results suggest the possibility of creating microelectronic devices that use a laser beam to erase and rewrite bits of information in materials engineered for random-access memory and data storage.
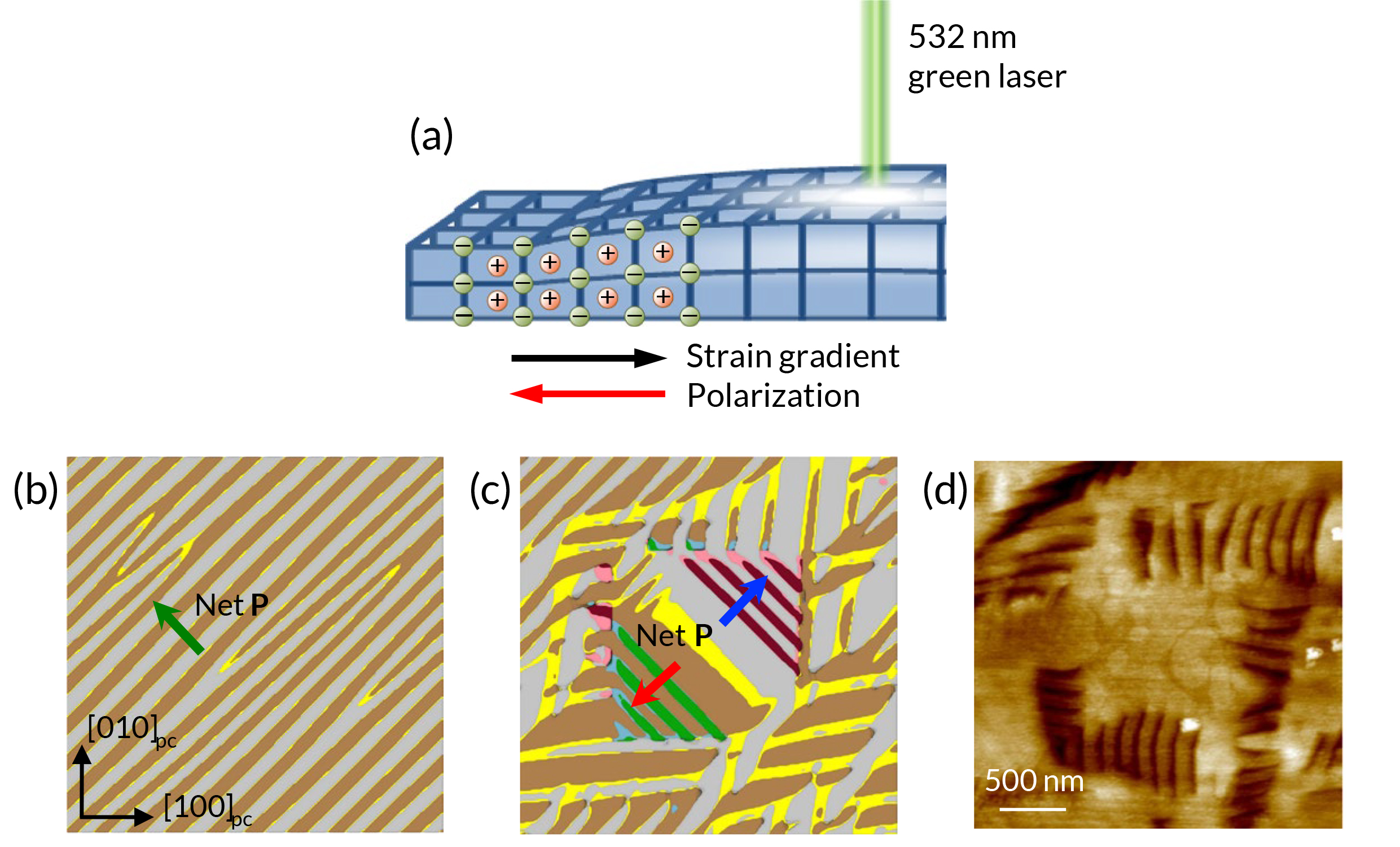
The multiferroic frontier
Many semiconductor-based devices use electric currents to control and manipulate bits of information encoded into tiny magnetic domains. However, this approach is reaching the physical limits of thermally stable feature sizes, and scientists are actively searching for the next generation of materials and processes that could lead to smaller, faster, more powerful devices.
One possible path forward has been opened up by the emergence of materials that can be engineered, layer by layer, to theoretical specifications. Multiferroics, for example, are designed materials with technologically useful properties that can be controlled by external fields. While many studies have been performed on the effects of electric and magnetic fields on multiferroics, very few studies have explored the use of optical modulation (i.e., laser light) as a way to tune magnetic and electronic ordering in such materials.
Bismuth ferrite under strain
Bismuth ferrite (BiFeO3) is a highly promising room-temperature multiferroic material. In thin-film form, BiFeO3 exhibits a significant spontaneous electrical polarization (ferroelectricity), which coexists with and is strongly coupled to the material’s antiferromagnetic order. Furthermore, when subjected to in-plane compressive strain, BiFeO3 undergoes a transition in crystal symmetry from a tetragonal phase (T-BFO) to a rhombohedral one (R-BFO). This shift induces modulations in functional properties, including conductivity, piezoelectricity, and refractive index. Under specific conditions, a “mixed-phase” sample of BiFeO3 can be on the verge of transitioning between T-BFO and R-BFO phases.
In images of mixed-phase BiFeO3 obtained using piezoresponse force microscopy (PFM), the phases appear as dark (R-BFO) and light (T-BFO) areas. After illumination by a 532 nm green laser, PFM images show a clear redistribution of the phases. Raman spectroscopy verified that local heating by the laser was responsible for the changes, and phase-field simulations accurately modeled the results.
Correlated ferroic orders
To probe the correlation between the ferroelectric and antiferromagnetic order in laser-illuminated BiFeO3, researchers used photoemission electron microcopy (PEEM) at ALS Beamline 11.0.1.1. The high-brightness, soft x-ray beamline allows straightforward switching between linearly and circularly polarized x-rays at the iron L-edges, enabling the researchers to map the ferroelectric and antiferromagnetic order in specific regions using linear and circular dichroism. The measurements, taken as a whole, reveal that tuning BiFeO3 domain structures via laser light simultaneously controls the correlated ferroelectricity, antiferromagnetism, and remanent magnetization.
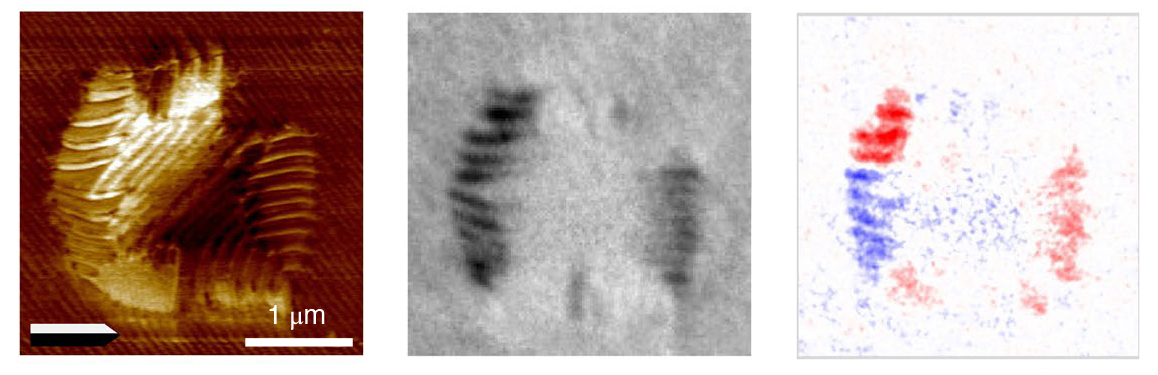
Rewriting the future
Finally, the researchers demonstrated that, simply by moving the laser spot, domain structures can be repeatably erased and rewritten. PFM images taken after illumination with a moving spot showed the evolution from pure T-BFO to the mixed phase and then back to pure T-BFO.
Overall, the work demonstrates nonvolatile, deterministic, local control of a multiferroic material by means of a laser at room-temperature conditions. This control simultaneously affects functional properties such as ferroelectricity and antiferromagnetism, and can leverage recent cutting-edge industrial efforts toward heat-assisted magnetic recording. Not only is it an effective approach to tailoring ferroic orders in complex materials, it also represents a distinct step toward technologically important microelectronic applications, such as nonvolatile random-access memories and data-storage devices.
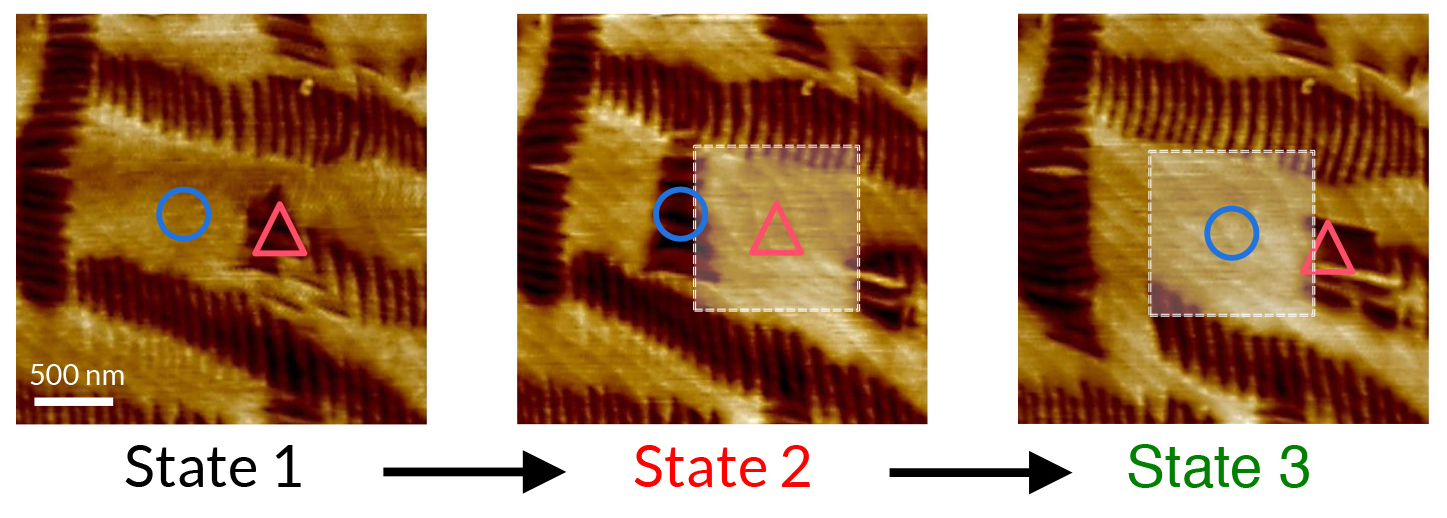
Contact: Rajesh Chopdekar
Researchers: Y.-D. Liou, Y.-Y. Chiu, Y.-C. Wu, Y.-C. Chen, and J.-C. Yang (National Cheng Kung University, Taiwan); R.T. Hart and Y. Cao (University of Texas at Arlington); C.-Y. Kuo (Max-Planck Institute for Chemical Physics of Solids, Germany, and National Synchrotron Radiation Research Center, Taiwan); Y.-L. Huang (National Chiao Tung University, Taiwan); R.V. Chopdekar (ALS); H.-J. Liu (National Chung Hsing University, Taiwan); A. Tanaka (Hiroshima University, Japan); C.-T. Chen (National Synchrotron Radiation Research Center, Taiwan); C.-F. Chang and L.H. Tjeng (Max-Planck Institute for Chemical Physics of Solids, Germany); V. Nagarajan (University of New South Wales, Australia); Y.-H. Chu (National Chiao Tung University and Academia Sinica, Taiwan).
Funding: Ministry of Science and Technology, Taiwan; Academia Sinica, Taiwan; National Chiao Tung University, Taiwan; University of Texas at Austin; Max Planck–Pohang University of Science and Technology/Hsinchu Center for Complex Phase Materials; and Australian Research Council Discovery Project. Operation of the ALS is supported by the U.S. Department of Energy, Office of Science, Basic Energy Sciences Program.
Publication: Y.-D. Liou, Y.-Y. Chiu, R.T. Hart, C.-Y. Kuo, Y.-L. Huang, Y.-C. Wu, R.V. Chopdekar, H.-J. Liu, A. Tanaka, C.-T. Chen, C.-F. Chang, L.H. Tjeng, Y. Cao, V. Nagarajan, Y.-H. Chu, Y.-C. Chen, and J.-C. Yang, “Deterministic optical control of room temperature multiferroicity in BiFeO3 thin films,” Nat. Mater. 18, 580 (2019), doi:10.1038/s41563-019-0348-x.
ALS SCIENCE HIGHLIGHT #401