SCIENTIFIC ACHIEVEMENT
Researchers used the Advanced Light Source (ALS) to study binding phases in Roman architectural concrete, revealing reactions and profound transformations that contribute to the material’s long-term cohesion and durability.
SIGNIFICANCE AND IMPACT
The findings add to our growing understanding of cementing processes in Roman concretes, informing resilient materials of the future.
Mysteries of Roman concrete’s durability
Marie Jackson, a research associate professor at the University of Utah, has devoted much of her career to understanding the scientific mysteries underlying the exceptional durability of Roman concretes. The ALS has been essential to her and her colleagues’ studies, helping to reveal the chemical and microstructural evolution of the materials.
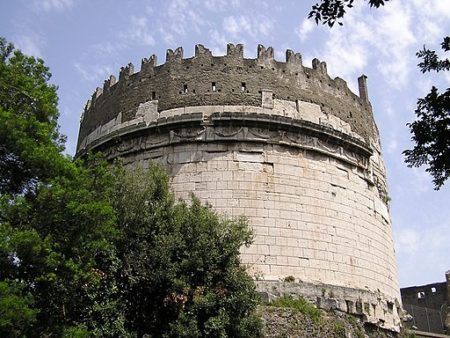
Concrete is made of rock aggregates and a binder. Modern concretes typically use Portland cement—made by burning a mixture of limestone and clay at high temperature—as binder. Roman concretes, in contrast, consist of coarse volcanic rock (or brick) aggregate bound with mortar made from hydrated lime and reactive tephra—the particles ejected from explosive volcanic eruptions.
In this study, Jackson, along with collaborators Admir Masic and Linda Seymour of the Massachusetts Institute of Technology and Nobumichi Tamura of the ALS, examined mortar samples from the Tomb of Caecilia Metella in Rome. The team hoped that the 2,050-year-old monument would provide insights into how Roman builders’ selections of reactive volcanic rock influenced the material characteristics of the very robust concrete.
X-rays probe the concrete’s structure
The researchers carefully removed samples from the tomb’s substructure with the assistance of the archaeologist at the monument. They then studied the samples with powder x-ray diffraction and prepared 30-micron-thick mortar slices, which they used to identify regions of interest with scanning electron microscopy.
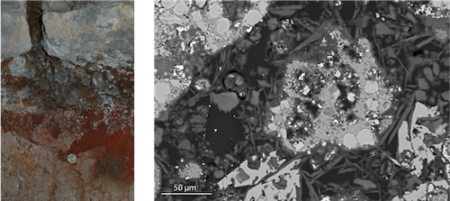
Next, they analyzed the thin-sectioned samples using x-ray microdiffraction and microfluorescence at Beamline 12.3.2, creating maps of the binding matrix and volcanic aggregate at 10-micron steps. They processed the data using the XMAS software developed by Tamura. The diverse phases in the mortar made it challenging to select experimental parameters that would cover the necessary range of d-spacing reflections. However, the extremely bright, energetic, and tightly focused ALS x-ray beam was well suited to penetrate the entire thickness of the sample, helping to reveal the highly heterogeneous and complex mixture of crystalline and nanocrystalline binding phases in the mortar.
An unexpected finding
As expected, the team found strätlingite crystals at the edges of volcanic aggregate particles—similar to their previous studies of Markets of Trajan concretes. The platy crystals provide obstacles to microcrack propagation. Unexpectedly, however, they found that the binding phase, composed of gel-like calcium-aluminum-silicate-hydrate (C-A-S-H), had undergone deep chemical and microstructural reorganization. Delicate wisps and tendrils had formed at the perimeters of intact C-A-S-H domains, some of which were revealed through x-ray microdiffraction to have a nanocrystalline preferred orientation—evidence of a long-term beneficial transformation of the material. Other domains had split into elongate features.
These profound changes in the C-A-S-H structure, which have not been previously described, suggest that when leucite crystals in the volcanic aggregate dissolved over time, their potassium was incorporated in the C-A-S-H binder. The wisps, tendrils, and elongate features further reinforce cohesion in the mortar, contributing to the chemical and mechanical resilience of the concrete.
Moving forward, the researchers want to learn more about the divergent reaction pathways that produce strätlingite crystals in some Roman architectural mortars and deep changes within the C-A-S-H binding phase in others. A better understanding of these processes could hold the secret to developing innovative, more durable concrete structures of the future.
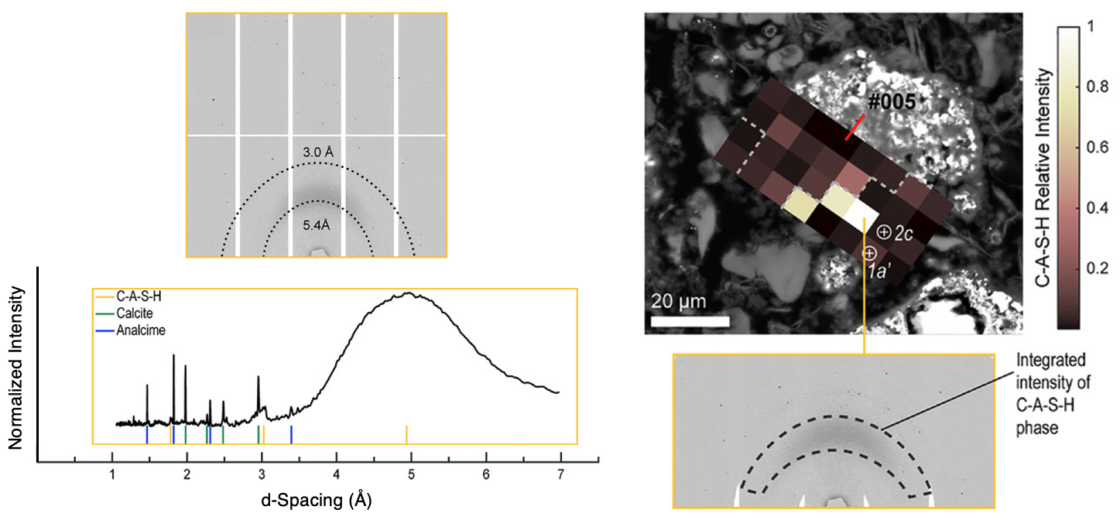
Contacts: Marie Jackson and Linda Seymour
Researchers: L.M. Seymour and A. Masic (Massachusetts Institute of Technology); N. Tamura (ALS); and M.D. Jackson (Univ. of Utah).
Funding: U.S. Department of Energy (DOE) Advanced Research Projects Agency-Energy (ARPA-E). Operation of the ALS is supported by the U.S. Department of Energy, Office of Science, Basic Energy Sciences program (DOE BES).
Publication: L.M. Seymour, N. Tamura, M.D. Jackson, and A. Masic, “Reactive binder and aggregate interfacial zones in the mortar of Tomb of Caecilia Metella concrete, 1C BCE, Rome,” J. Am. Ceram. Soc. 105, 1503 (2021), doi:10.1111/jace.18133.
ALS SCIENCE HIGHLIGHT #456