Rechargeable lithium-ion batteries power most portable electronics and are becoming more widely used in large-scale applications like electric vehicles. Scientists have long observed that lithium iron phosphate nanoparticles are one of the best performing battery electrode materials, able to repeatedly charge and discharge in an extremely reversible manner, but the precise mechanism responsible for their performance has remained unclear. By using a powerful suite of imaging tools, a team of scientists at the ALS, the University of Illinois at Chicago (UIC), and the NorthEast Center for Chemical Energy Storage has revealed a relationship between the chemical processes that drive a battery’s operation and the mechanical degradation of the electrode material. Their findings show that small crystal size is key to maintaining mechanical stability upon cycling, and establish soft x-ray ptychography as an essential tool for studying chemical states in nanoparticles.
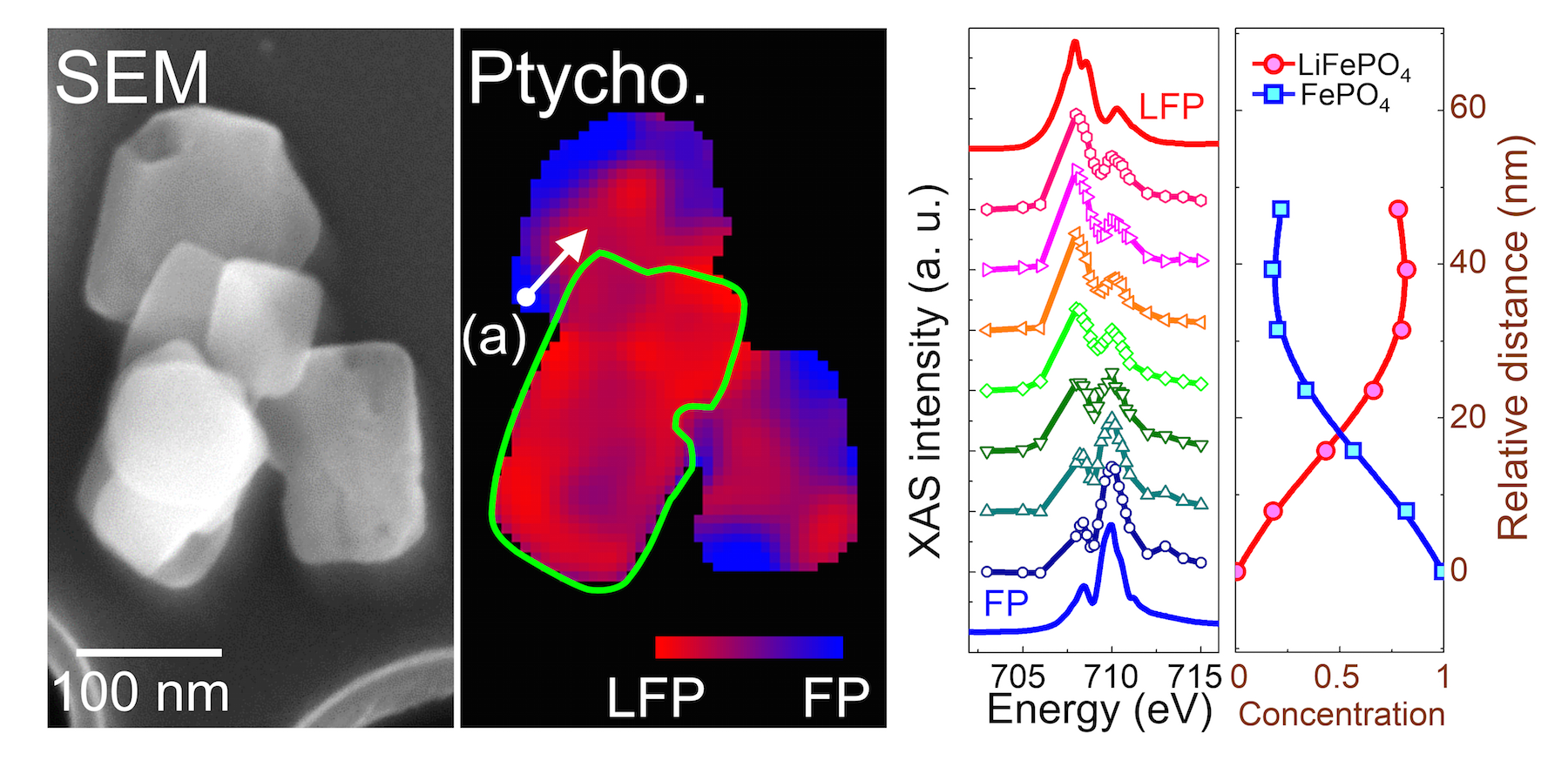
Batteries operate through the movement of charged particles between two electrode materials. In the case of lithium-ion batteries, the electrodes are typically made of graphite for the anode and a compound like lithium iron phosphate (LiFePO4) for the cathode. As the battery charges, lithium ions and electrons move from the LiFePO4 to the graphite—a process called delithiation. The process must reverse during discharge to ensure long battery life. As the battery discharges and recharges, the electrodes’ crystal lattices expand and shrink in response to the gain and loss of ions, creating strain in the structure that fatigues, and ultimately fractures, the material. To determine precisely how the evolution of composition and microstructure varies with crystal size and shape requires spatial and chemical resolution at the nanoscale.
Ptychography uses a combination of multiple coherent diffraction measurements to construct 2D or 3D spatial maps with high resolution and chemical sensitivity. Because of the sensitivity of soft x-rays to electronic states, they can be used in ptychography to image chemical phase transformations and the resulting mechanical consequences. The ability to map these changes at the required nanoscale resolution without damaging the sample material is a capability unique to the ALS, as featured in a previous ALS highlight.
After synthesizing and partially delithiating LiFePO4 crystals of different sizes, ALS and UIC researchers used soft x-ray ptychographic microscopy, combined with x-ray absorption spectroscopy and electron microscopy, to map chemical and structural changes in the micro- and nanocrystals during the delithiation process. Ptychography measurements were recorded with the STXM instruments at ALS Beamlines 11.0.2 and 5.3.2.1.
The results showed that all sizes of partially delithiated crystals contained two phases—the original LiFePO4 and its delithiated state, FePO4. The high resolution of the imaging technique also allowed the team to see the location of undesirable cracks in the crystal structure that can degrade the material’s performance as an electrode. They found that the defects tended to be located along the interface where the two phases meet, which is also where the crystal structure experiences the most strain as lattices of different sizes attempt to match up. More significantly, the team found that the larger microcrystals were severely fractured, whereas the nanocrystals had no mechanical damage, which is consistent with the prediction that the number of potential defects in a crystal decreases significantly with a reduction in its size.
The findings indicate that mechanics are a principal driver in the outstanding electrode performance of LiFePO4 nanoparticles. They also demonstrate the importance of carefully controlling morphological and chemical characteristics of electrode materials in next-generation electrical energy storage systems, which will help achieve theoretical limits of energy density and battery lifetime.
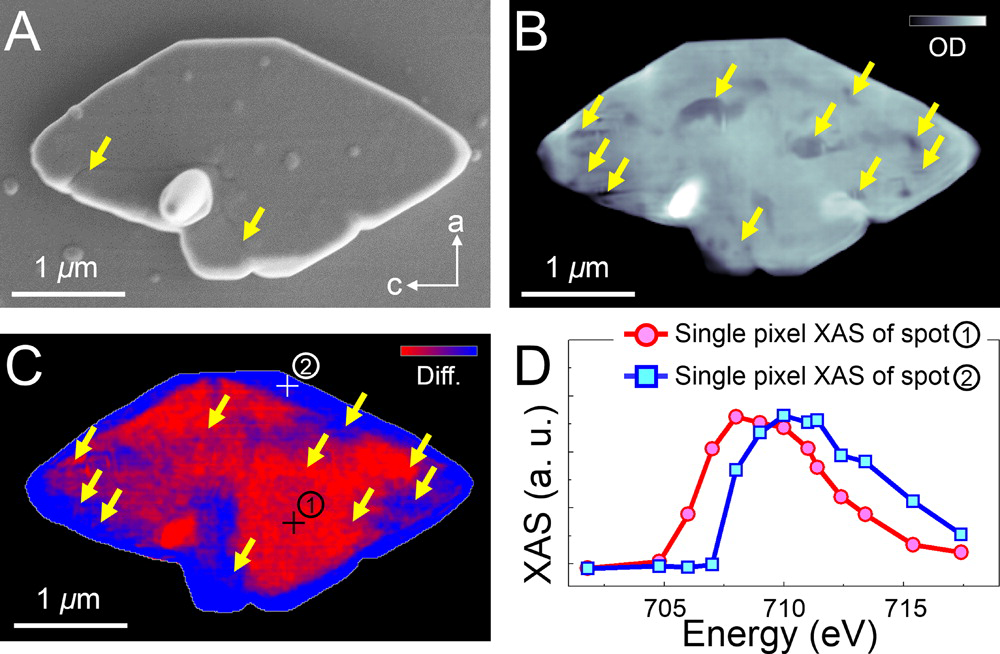
Contact: David Shapiro
Research conducted by: Y.-S. Yu (ALS, U. of Illinois at Chicago, Berkeley Lab, and UC San Diego); C. Kim and J. Carbana (U. of Illinois at Chicago); D. Shapiro, M. Farmand, T. Tyliszczak, A.L.D. Kilcoyne, R. Celestre, S. Marchesini, P. Denes, T. Warwick, and H. Padmore (ALS); D. Qian and Y.S. Meng (UC San Diego); J. Joseph and R. Kostecki (Berkeley Lab); F. C. Strobridge (U. of Cambridge); and C.P. Grey (U. Cambridge and Stony Brook U.).
Research funding: U.S. Department of Energy (DOE), Office of Basic Energy Sciences (BES) and Office of Advanced Scientific Computing Research (ASRC). Operation of the ALS is supported by DOE BES.
Publication about this research: Y.-S. Yu, C. Kim, D. Shapiro, M. Farmand, D. Qian, T. Tyliszczak, A.L.D. Kilcoyne, R. Celestre, S. Marchesini, J. Joseph, P. Denes, T. Warwick, F.C. Strobridge, C.P. Grey, H. Padmore, Y.S. Meng, R. Kostecki, and J. Cabana, “Dependence on crystal size of the nanoscale chemical phase distribution and fracture in LixFePO4,” Nano Lett. 15, 4282 (2015).
ALS SCIENCE HIGHLIGHT #319