SCIENTIFIC ACHIEVEMENT
Multimodal probes at the Advanced Light Source (ALS) and the Molecular Foundry revealed a way to prevent the formation of undesirable phases in a perovskite-type compound that shows promise for the efficient harvesting of light for solar cells.
SIGNIFICANCE AND IMPACT
The work led to new fabrication protocols that resulted in devices with improved power-conversion efficiencies and operational stability.
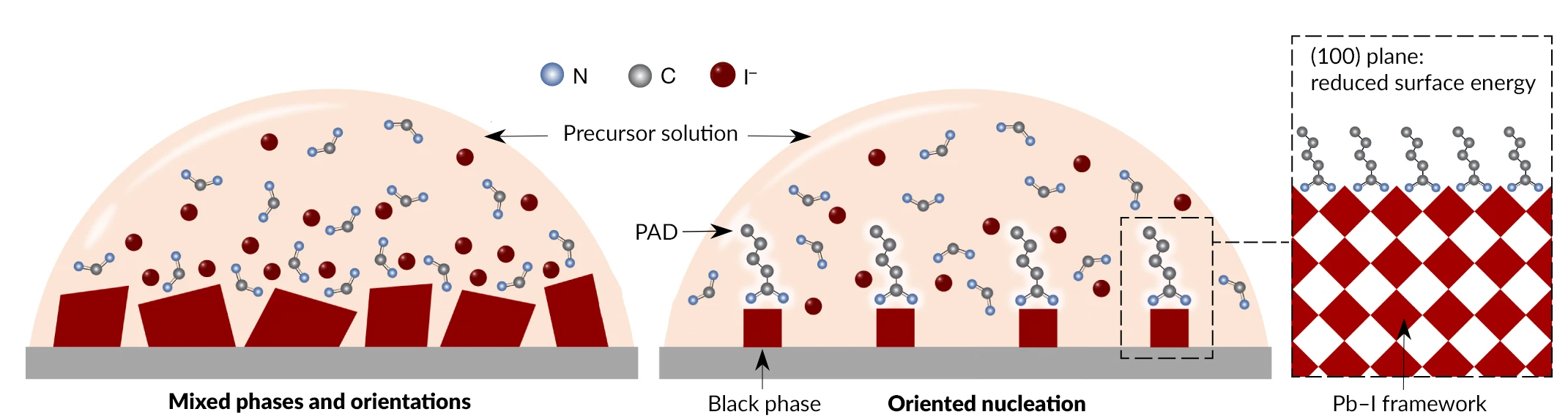
A new class of PV materials
The harnessing of solar power is a critical pillar in the next generation of electrical infrastructure, and it will require large-scale manufacture and deployment of photovoltaic (PV) panels. A relatively new class of materials, organic–inorganic halide perovskites, offers an alternative to silicon for PV power generation.
Halide perovskites are prime candidates for research because they offer a range of benefits, including being up to 1000 times thinner than silicon, absorbing incoming solar irradiation more efficiently, and having a better matched bandgap for the solar spectrum. Perovskite thin-film fabrication also offers lower-cost processes, but more research, such as this study, needs to be done to capitalize on that potential.
Polytypes—black and yellow phases
Formamidinium lead iodide (FAPbI3) is one of the most promising halide perovskite candidates for PV applications. One challenge, however, is the existence of polytypes of FAPbI3 (different configurations of the same atoms), called black and yellow phases. At room temperature, the yellow phase is the most stable, but it is relatively transparent to the solar spectrum. In contrast, the black phase absorbs most of the solar spectrum, but it is energetically unfavorable and therefore not normally stable at room temperature. As a consequence, the photoactive black phase is hampered by conversion to the non-photoactive yellow phase. To counteract this, researchers have developed a new method of perovskite crystallization that reliably suppresses yellow-phase formation by encouraging the oriented nucleation of FAPbI3 crystals.
Multimodal crossover capabilities
The crystallization of FAPbI3 is a rapid process, making it difficult to monitor in real time. To overcome this challenge, the researchers made use of significant crossover between the expertise and capabilities available at Berkeley Lab’s Molecular Foundry and the ALS.
At ALS Beamline 12.3.2, x-ray microdiffraction revealed key features of the crystal structure in real time, as it developed from precursor solutions. This required building a spin coater that worked within the beamline’s hutch. While the film was fabricated, diffraction and photoluminescence data could be collected simultaneously. Sample preparation and complementary measurements were performed at the Foundry. With these capabilities, the researchers were able to monitor crystal phase changes and study the influence of additives on the crystallization pathway.
PAD additive success
The results showed that when pentanamidine hydrochloride (PAD) was added to the starting materials, the yellow phase and other intermediate phases were suppressed. According to the researchers’ analysis, PAD leads to a decrease in surface energy for the (100) plane, driving the preferential formation of (100)-oriented black-phase FAPbI3.
PAD also allowed for more synthesis control by slowing down the crystallization process, and the well-oriented growth produced crystals of higher quality. Devices made using the PAD-altered process demonstrated consistently higher power-conversion efficiency and considerably improved operational stability. The additive strategy was applied to different fabrication processes and was found to be universally applicable as a strategy to achieve stable black-phase perovskite.
In the future, the researchers hope to expand these advanced characterization capabilities to industry-relevant and scalable processes, as well as broadening the scope to other material classes beyond perovskites, such as organic semiconductors and other functional materials.
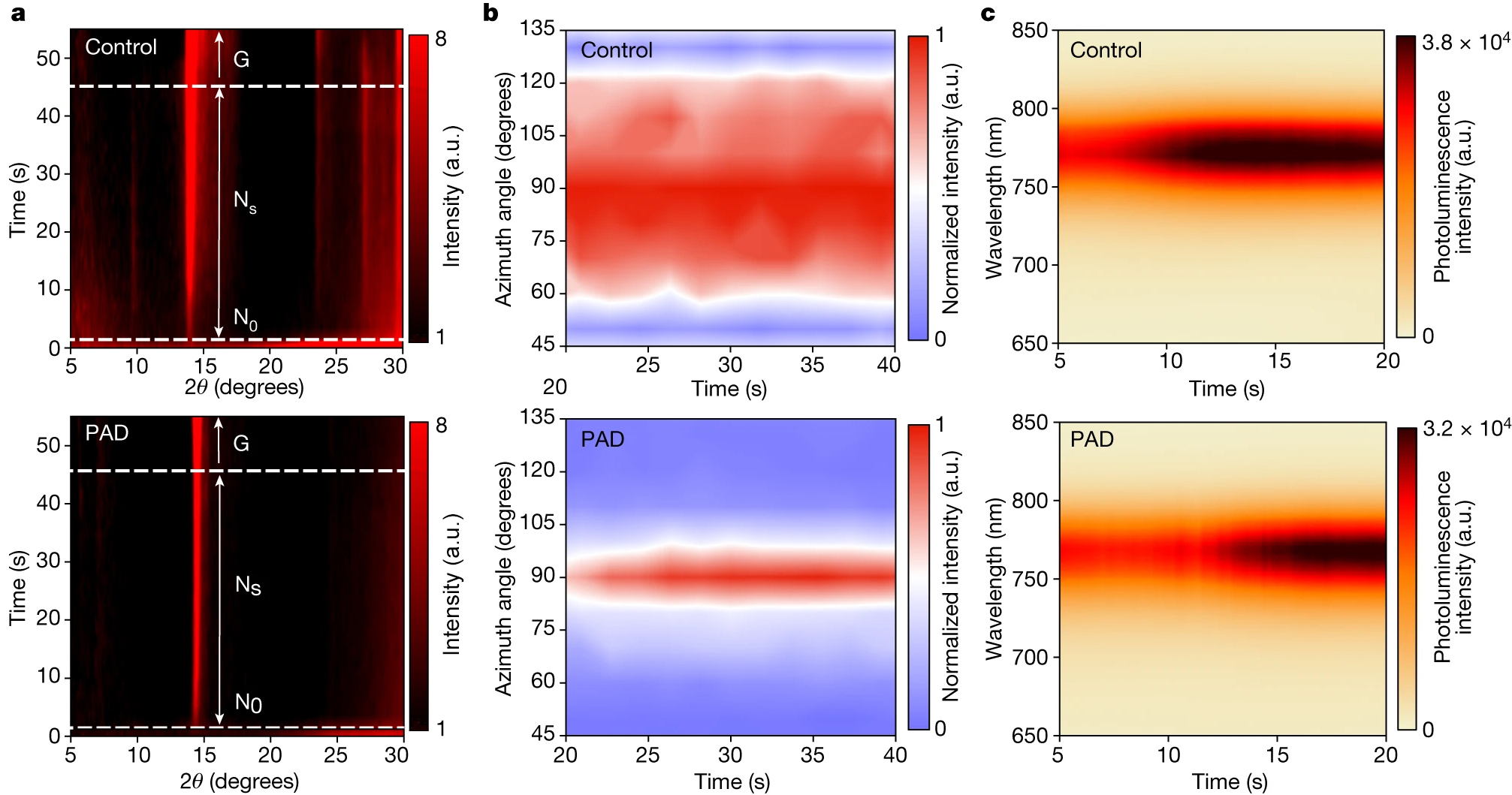
Contact: Carolin Sutter-Fella
Researchers: P. Shi, W. Fan, Y. Tian, and K. Zhao (Zhejiang Univ. and Westlake Univ., China); Y. Ding (École Polytechnique Fédérale de Lausanne [EPFL], Switzerland, and North China Electric Power Univ.); B. Ding, P.J. Dyson, and M.K. Nazeeruddin (EPFL); Q. Xing, S. Tan, and Y. Yang (Univ. of California, Los Angeles); D. Yang and J. Xue (Zhejiang Univ., China); T. Kodalle and C.M. Sutter-Fella (Berkeley Lab); I. Yavuz (Marmara Univ., Turkey); C. Yao (Fudan Univ., China); J. Xu, D. Gu, X. Zhang, L. Yao, and R. Wang (Westlake Univ., China); and J.L. Slack (ALS).
Funding: Natural Science Foundation of Zhejiang Province of China, National Natural Science Foundation of China, Shanxi-Zheda Institute of Advanced Materials and Chemical Engineering (China), Westlake University (China), Valais Energy Demonstrators fund (EPFL), German Research Foundation, and National Center for High Performance Computing of Turkey. Operation of the ALS and Molecular Foundry is supported by the US Department of Energy, Office of Science, Basic Energy Sciences program.
Publication: P. Shi, Y. Ding, B. Ding, Q. Xing, T. Kodalle, C.M. Sutter-Fella, I. Yavuz, C. Yao, W. Fan, J. Xu, Y. Tian, D. Gu. K. Zhao, S. Tan, X. Zhang, L. Yao, P.J. Dyson, J.L. Slack, D. Yang, J. Xue, M. Khaja Nazeeruddi, Y. Yang, and R. Wang, “Oriented nucleation in formamidinium perovskite for photovoltaics,” Nature 620, 323 (2023), doi:10.1038/s41586-023-06208-z.
Adapted from the Molecular Foundry article, “Towards Longer-Lived Solar Cells: Coaxing Molecules to Stand Tall.”
ALS SCIENCE HIGHLIGHT #487