As coral colonies thrive, they build strong, sculptural exoskeletons on which they live. How will ocean acidification affect these living works of art? Researchers have recently discovered some good news: coral skeletons grow by the aggregation of surprisingly large particles that form within the coral’s living tissue, where acidity can be controlled biologically. The evidence was provided by photoemission electron microscopy (PEEM) at the ALS, an x-ray technique that can map the chemical and physical characteristics of materials on a microscopic scale. The discovery has ramifications, not only for the health of coral reefs, but for applications such as 3D printing as well.
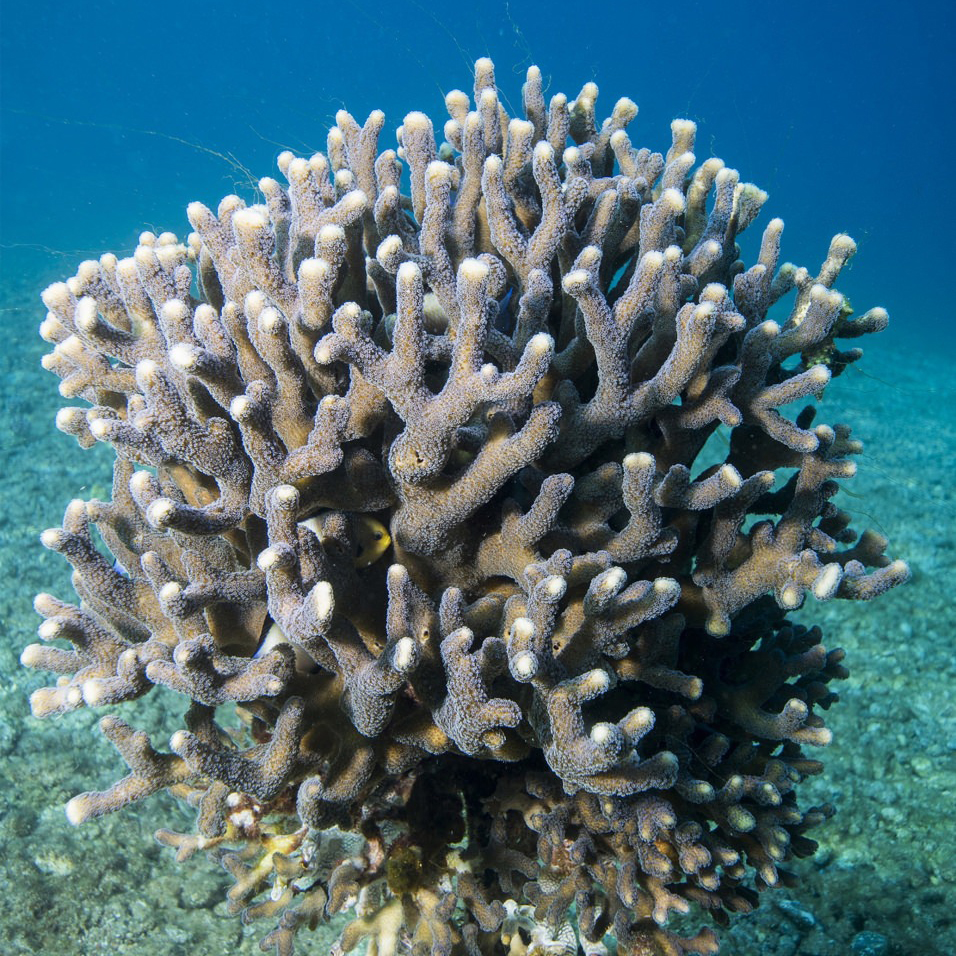
The type of coral studied in this work, Stylophora pistillata, is a very common, fast-growing coral found in tropical waters around the world. It is composed of colonies of small, sea-anemone-like creatures (“polyps”) that are rooted to a surface on one end and feed from the other. The polyps are supported by hard, rock-like skeletons of aragonite—crystals of calcium carbonate (CaCO3)—that the polyps themselves deposit. However, the mechanism by which skeletal growth occurs has been the subject of heated debate.
The classical growth model assumes that ions from seawater are transported through the polyp and attach to the growing skeleton surface one ion at a time, to directly form aragonite crystals. An alternative view holds that noncrystalline, amorphous calcium carbonate (ACC) precursor particles form inside the polyp, are deposited on the skeleton, and much later crystallize to aragonite. Because the ACC is formed inside the polyp, it would be isolated from changes in ocean conditions. Once attached to the skeleton surface, the amorphous particles crystallize into aragonite (a thermodynamically “downhill” transition) after about 30 hours.
Can such amorphous precursors be observed before crystallization occurs? The researchers answered using PEEM experiments at ALS Beamline 11.0.1.1. The PEEM instrument enabled the production of high-resolution component maps showing where various forms of CaCO3 were located in freshly sacrificed coral samples. In a stack of PEEM images acquired while scanning the x-ray energy across the Ca L-edge, each pixel has the full x-ray absorption spectrum, which identifies the relative abundances CaCO3 components at that spot. Each component was assigned a color (red, green, or blue), and the pixel was colored accordingly. The results showed clear evidence of two amorphous precursors: hydrated ACC (ACC-H2O) and anhydrous ACC.
Moreover, Beamline 11.0.1.1’s polarization control allowed measurement of the crystal orientations (and thus the sizes) of the particle grains once the precursors had crystallized. The grains turned out to be about 400 nm in size—much bigger than the tens of nanometers expected. These results were also confirmed with microdiffraction experiments at Beamline 12.3.2.
The researchers concluded that the deposition of large ACC particles explains S. pistillata’s fast growth rate—more than 100 times faster than would be expected for ion-by-ion precipitation from solution. Subsequent studies done to synthetically grow aragonite ion-by-ion to compare growth rates showed, surprisingly, that particle-by-particle growth of aragonite also occurs in vitro, in the absence of any organic molecules. The researchers note that this mechanism for quickly growing a hard, space-filling material is precisely what 3D printing is designed to do. They suggest that, in principle, it might be possible to 3D print bone implants or other devices for industrial or medical purposes, much faster—one large particle at a time rather than one ion (or one atom) at a time.
Future directions for this line of research include using PEEM to look at different types of coral, including species that live in much deeper, colder water, with different morphologies. The researchers would like to determine whether such species grow in the same manner as S. pistillata and if they do, what common characteristics in these diverse organisms give them this capability.
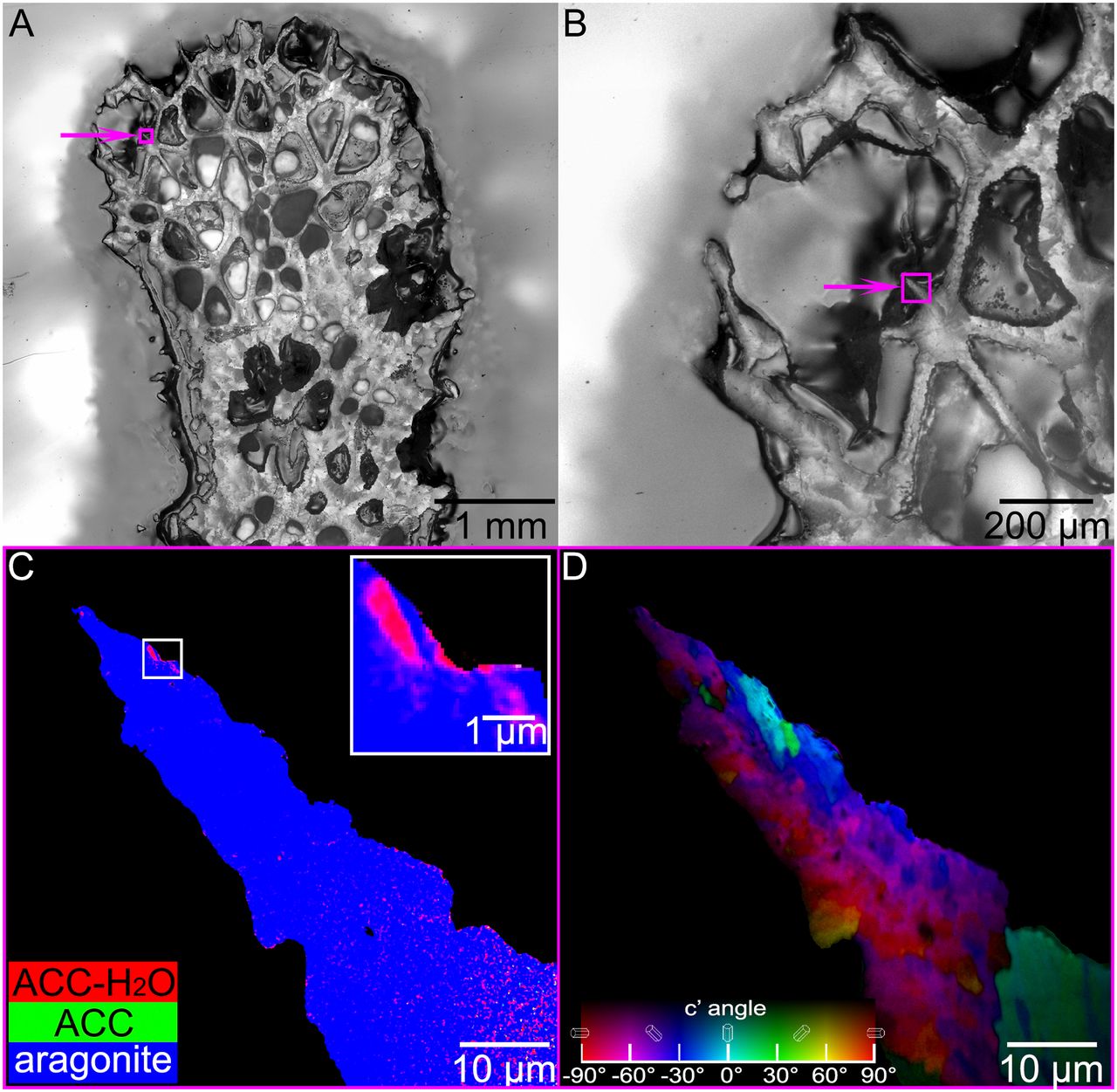
Contact: Pupa Gilbert
Research led by P.U.P.A. Gilbert and conducted by: T. Mass (U. of Haifa, Israel); A.J. Giuffre, C.-Y. Sun, C.A. Stifler, M.J. Frazier, and P.A. Gilbert (U. of Wisconsin–Madison); M. Neder (U. of Haifa and Interuniversity Institute for Marine Sciences–Eilat, Israel); and N. Tamura, C.V. Stan, and M.A. Marcus (ALS).
Research funding: U.S. Department of Energy, Office of Science, Basic Energy Sciences Program (DOE BES); National Science Foundation; United States–Israel Binational Science Foundation; and Israel Science Foundation. Operation of the ALS is supported by DOE BES.
Publication about this research: T. Mass, A.J. Giuffre, C.-Y. Sun, C.A. Stifler, M.J. Frazier, M. Neder, N. Tamura, C.V. Stan, M.A. Marcus, and P.U.P.A. Gilbert, “Amorphous calcium carbonate particles form coral skeletons,” PNAS 114, E7670 (2017). doi:10.1073/pnas.1707890114
For further information, see This Week in Science, “A protoparticular path to corals,” and Univ. of Wisconsin press release, “Coral skeletons may resist the effects of acidifying oceans.”
ALS SCIENCE HIGHLIGHT #364