Immortality is not a good thing for cells, and in fact, cells will destroy themselves in a process called apoptosis when they are a danger to other cells. For instance, when a cell is infected by a virus it becomes an unwilling factory for the virus, which uses the cell machinery to produce ever more copies of itself. Eventually, if the cell doesn’t die, it will spew all those new viruses into the bloodstream. The process of apoptosis spares other cells this same fate.
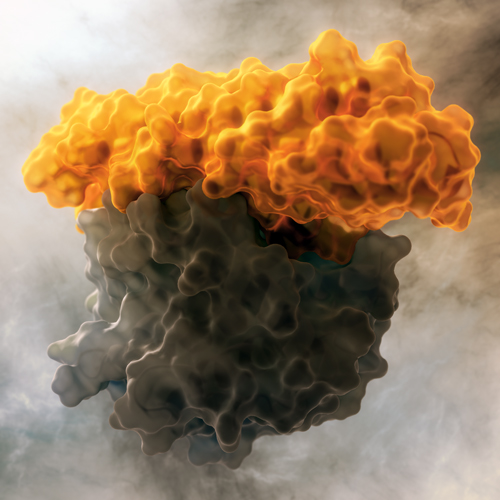
Some viruses plan for this, and produce proteins to stop apoptosis, like creating “zombie cells” which are not truly alive, but exist only to infect others. The Epstein-Barr virus (EBV) is one of those zombie-cell producing viruses. Another negative outcome of stopping apoptosis is that keeping cells alive beyond their healthy point can contribute to the development of cancer. EBV was one of the first viruses to be identified in association with human cancer, but it is not the only one.
Keeping cells alive requires a delicate balancing act between proteins that promote apoptosis (executioners) and proteins that inhibit it (survivalists), as well as other proteins that inhibit the executioners, and still other proteins that enhance the survivalists. EBV produces multiple proteins to prevent apoptosis, including BHRF1 to sequester and inhibit the executioners, leading to cell survival even when the cell is infected.
The researchers in this study wanted to design a protein to inhibit the inhibitor (BHRF1). The idea was to design a protein to recognize and bind BHRF1, in order to restore apoptosis. The first step was determining the specific sequence of amino acids on the executioner protein that bind BHRF1. This “folding nucleus” was then grafted into a larger helical bundle, which served as the scaffold. The trick was to build a helical bundle of the correct geometry around this folding nucleus by piecing together fragments from naturally occurring proteins. Residues around the graft site were also mutated to minimize the binding energy, and thereby increase the affinity, between BHRF1 and the designer proteins. Even tighter affinity was achieved by “directed evolution” of the scaffolding structure itself to make it more rigid, essentially locking it in the correct geometry for target interactions. The researchers designed thousands of such proteins and analyzed them for stability and projected binding affinity, and then selected less than a hundred of the top proteins for further analysis. A small number of the top proteins were expressed and purified from E. coli, and further binding tests selected two proteins that bound to BHRF1 with acceptable affinities. The top candidate was crystallized bound to BHRF1, and the structure solved at Beamline 5.0.2. This structure showed how the computational model of the designed protein did and did not match reality; small differences in loop areas which were not predicted by the computational modeling were an important part of the feedback process, because they inform future computational design. Finally, the top protein was tested against EBV-associated cancer and it held up well: the designed inhibitor not only triggered apoptosis in EBV cancer cell lines in the lab, but also when delivered with a carrier system in live animals, suppressed tumor growth and extended survival.
The design of proteins “de novo” is not a new field, but until recently designer proteins were rarely also functional. In this study, new design approaches were defined and then used successfully to develop a potential inhibitor of EBV-associated cancer. The study shows not just how to help defeat EBV, but also opens up a whole new way to design proteins against viruses and ultimately, cancer.
Contact: David Baker
Research conducted by: E. Procko, G.Y. Berguig, Y. Song, A.J. Convertine, Y. Cheng, and P.S. Stayton (University of Washington); B.W. Shen, S. Frayo, D. Margineantu, G. Booth, D.M. Hockenbery, O.W. Press, and B.L. Stoddard (Fred Hutchinson Cancer Research Center); B.E. Correia and W.R. Schief (The Scripps Research Institute); and David Baker (University of Washington, Howard Hughes Medical Institute).
Research funding: This work was supported by the NIH, NSF, Washington State Life Sciences Discovery Fund, and the Defense Threat Reduction Agency. Operation of the ALS is supported by DOE BES.
Publication about this research: E. Procko, G.Y. Berguig, B.W. Shen, Y. Song, S. Frayo, A.J. Convertine, D. Margineantu, G. Booth, B. E. Correia, Y. Cheng, W.R. Schief, D.M. Hockenbery, O.W. Press, B.L. Stoddard, P.S. Stayton, and D. Baker, “A Computationally Designed Inhibitor of an Epstein-Barr Viral Bcl-2 Protein Induces Apoptosis in Infected Cells,” Cell 157, 1644 (2014). doi:10.1016/j.cell.2014.04.034
ALS SCIENCE HIGHLIGHT #303