SCIENTIFIC ACHIEVEMENT
Electronic-structure studies at the Advanced Light Source (ALS) showed that both infinitely light and infinitely massive particles coexist in a material with a star-shaped (kagome) crystal lattice.
SIGNIFICANCE AND IMPACT
The material’s rich array of electronic behaviors shows promise for future spintronic applications and represents a new frontier for studying exotic phases of matter.
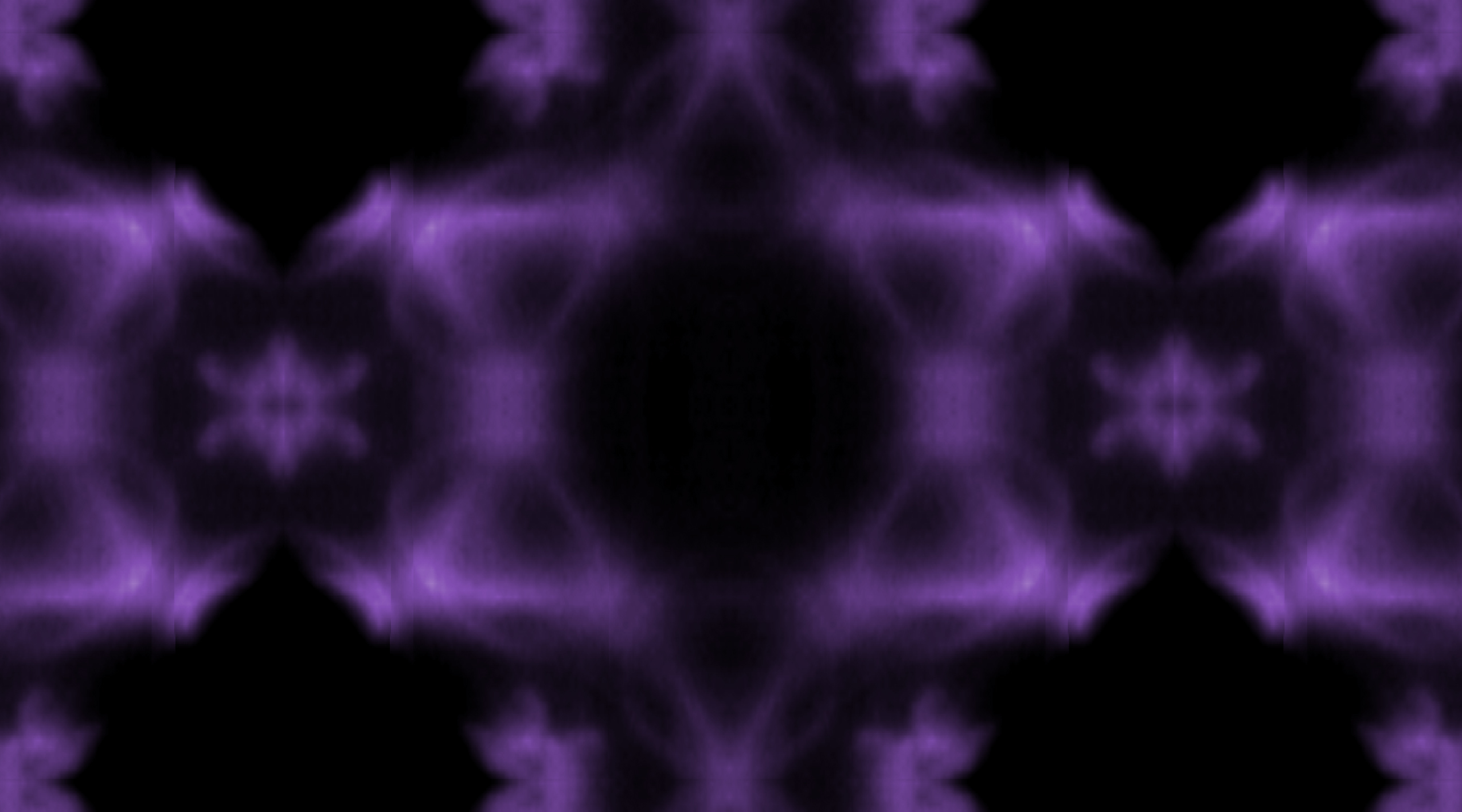
The significance of kagome
Iron (Fe) and tin (Sn) are common, unremarkable metals, but when their atoms are combined in certain patterns, unusual properties can emerge. The patterns, which resemble the kagome style of Japanese basket weaving, have important effects on electronic behavior. A well-known example of this is the emergence of Dirac fermions (i.e., electrons that behave as if they were massless) in the hexagonal lattice of graphene.
A kagome lattice is also a hexagonal network, with the addition of corner-sharing triangles around the hexagons. Electrons in kagome lattices have long been predicted to behave as if they were infinitely light (like Dirac fermions in graphene) or infinitely heavy (highly localized and confined). Materials with these properties plus the intrinsic magnetism of iron can exhibit topological and correlated electronic phenomena that could be useful in future device applications, including spintronic technologies.
In search of Dirac cones and flat bands
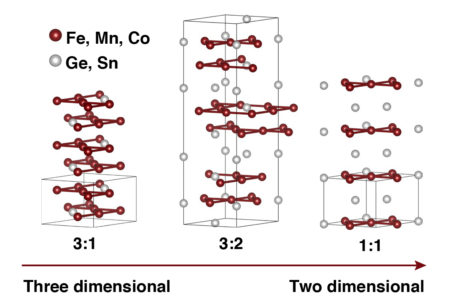
In band-structure diagrams, the presence of Dirac fermions is indicated by Dirac cones, and the signature of infinitely massive particles is a flat band. While both these features have been predicted by theoretical models of ideal kagome metals, their experimental realization has proven to be elusive. This is mainly due to the presence of complicating interactions, including those occurring between two-dimensional kagome layers stacked into three-dimensional materials.
Since 2016, a team of MIT researchers has focused on FemSnn compounds (m:n = 3:1, 3:2, 1:1) with different stacking structures. While a previous study on a 3:2 compound verified the presence of Dirac fermions, it did not find evidence of flat bands. A breakthrough came when they synthesized a 1:1 compound (FeSn) in which the kagome layers were well separated by a spacer layer of Sn.
MAESTRO probes individual layers
The researchers investigated the electronic structure of FeSn using two complementary techniques: angle-resolved photoemission spectroscopy (ARPES) at ALS Beamline 7.0.2 (MAESTRO, a light-based surface probe) and de Haas–van Alphen (dHvA) quantum oscillations (a bulk probe that uses high magnetic fields). One complexity in measuring the electronic structure of FeSn is that its surfaces expose two different layer terminations: one for the kagome layer and one for the spacer layer. These two domains are microscopically mixed on a cleaved surface. MAESTRO overcomes this by microfocusing the light beam below the size of the surface domains, measuring the electronic structure of each termination independently.
An ideal kagome metal
The experiments unambiguously demonstrated the simultaneous emergence of both Dirac fermions and flat bands in FeSn, as predicted for an ideal kagome metal. The results also revealed the presence of unexpected surface Dirac fermions on the spacer-layer terminations. Theoretical work demonstrated that the surface Dirac states, when combined with the antiferromagnetic spin textures of FeSn, are a rare example of fully spin-polarized Dirac fermions.
Because the kinetic energy of flat-band electrons is extremely low, electron-electron interactions become important. Thus, flat-band systems are ideal for realizing correlation-driven phenomena, including high-temperature superconductivity and anomalous magnetism. In the future, the researchers hope to tune the flat-band position toward the Fermi level, to see what kind of correlated phases can be produced based on kagome-driven flat bands.
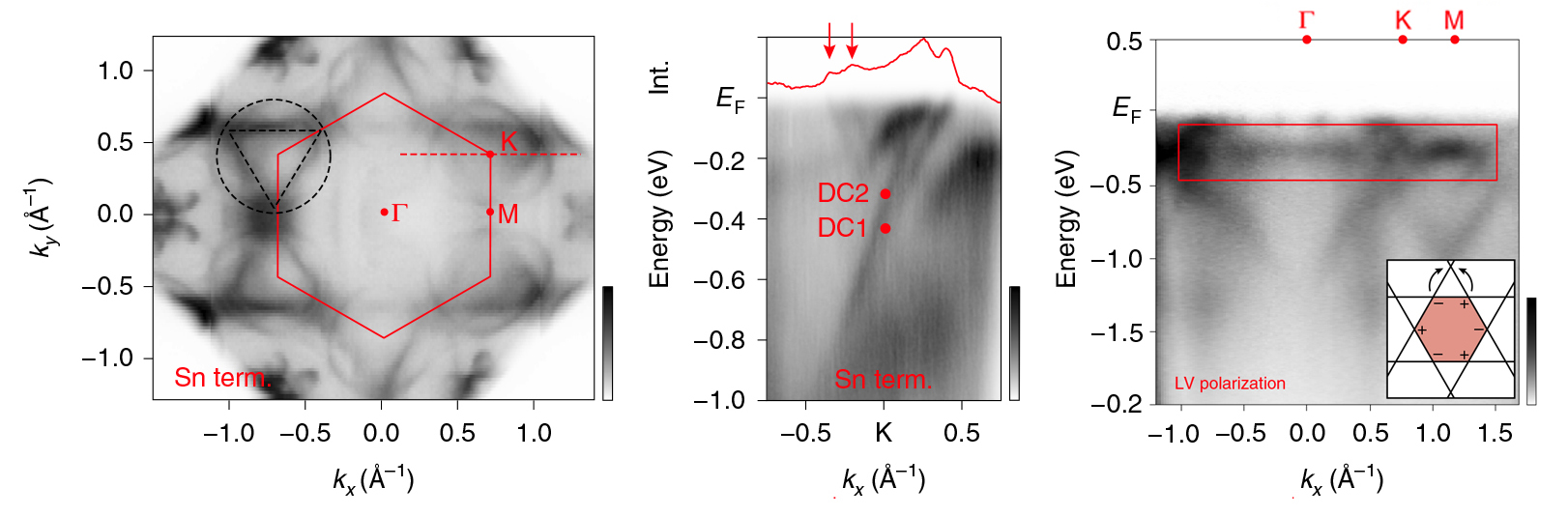
Contacts: Min Gu Kang, Riccardo Comin, and Joe Checkelsky
Researchers: M. Kang, L. Ye, A. Levitan, M. Han, J.G. Checkelsky, and R. Comin (Massachusetts Institute of Technology); S. Fang, D.C. Bell, and E. Kaxiras (Harvard University); J.-S. You, J.I. Facio, and J. van den Brink (Leibniz Institute for Solid State and Materials Research Dresden, Germany); C. Jozwiak, A. Bostwick, and E. Rotenberg (ALS); M.K. Chan and R.D. McDonald (National High Magnetic Field Laboratory, Los Alamos); D. Graf (National High Magnetic Field Laboratory, Tallahassee); K. Kaznatcheev and E. Vescovo (Brookhaven National Laboratory); M. Richter (Leibniz Institute for Solid State and Materials Research Dresden, and Dresden Center for Computational Materials Science, Germany); and M. Prasad Ghimire (Leibniz Institute for Solid State and Materials Research, Germany, and Tribhuvan University, Nepal).
Funding: Alfred P. Sloan Foundation, Gordon and Betty Moore Foundation, National Science Foundation, Samsung Foundation of Culture, Tsinghua Education Foundation, German Research Foundation, Alexander von Humboldt Foundation, Leibniz Institute for Solid State and Materials Research Dresden, State of Florida, and U.S. Department of Energy, Office of Science, Basic Energy Sciences Program (DOE BES). Operation of the ALS is supported by DOE BES.
Publication: M. Kang, L. Ye, S. Fang, J.-S. You, A. Levitan, M. Han, J.I. Facio, C. Jozwiak, A. Bostwick, E. Rotenberg, M.K. Chan, R.D. McDonald, D. Graf, K. Kaznatcheev, E. Vescovo, D.C. Bell, E. Kaxiras, J. van den Brink, M. Richter, M. Prasad Ghimire, J.G. Checkelsky, and R. Comin, “Dirac fermions and flat bands in the ideal kagome metal FeSn,” Nat. Mater. 19, 163 (2019), doi: 10.1038/s41563-019-0531-0.
ALS SCIENCE HIGHLIGHT #414