The energy economy of the future is likely to include hydrogen produced by renewable energy sources such as wind and solar. While intense efforts are focused on developing the technologies needed to produce hydrogen, the production side is only one of three legs necessary to support a complete hydrogen ecosystem. Once produced, hydrogen must also be transported to where it is used, and then it must be stored—for potentially months or years, depending on the application.
Developing solutions to the storage problem is the focus of the Hydrogen Materials Advanced Research Consortium (HyMARC), a team of five national laboratories funded by the Hydrogen Fuel Cell Technologies Office of the US Department of Energy (DOE). From HyMARC’s inception in 2015, a central objective has been to provide foundational scientific insight into the phenomena controlling hydrogen uptake and release by condensed-phase materials.
This video about the Hydrogen Materials Advanced Research Consortium was featured at the Materials Research Society 2019 Fall Meeting in Boston, Massachusetts. (Video credit: WebsEdge Science/Materials Research Society)
Recently, the HyMARC Approved Program (AP) at the Advanced Light Source (ALS) was renewed for the second time, underscoring the key role that soft x-ray techniques such as x-ray absorption spectroscopy (XAS), scanning transmission x-ray microscopy (STXM), and ambient-pressure x-ray photoelectron spectroscopy (APXPS) have played in unraveling some of the greatest challenges blocking discovery of hydrogen storage materials.
“Without the ALS, we wouldn’t be able to probe the chemistry that takes place at buried interfaces in hydrogen storage materials or understand how certain catalysts enable liquid compounds to absorb and release hydrogen,” said Mark Allendorf, the AP principal investigator and a co-director of HyMARC. “Using the ALS’s spectroscopy and microscopy tools, HyMARC has upended several established paradigms that have guided hydrogen storage research for decades.”
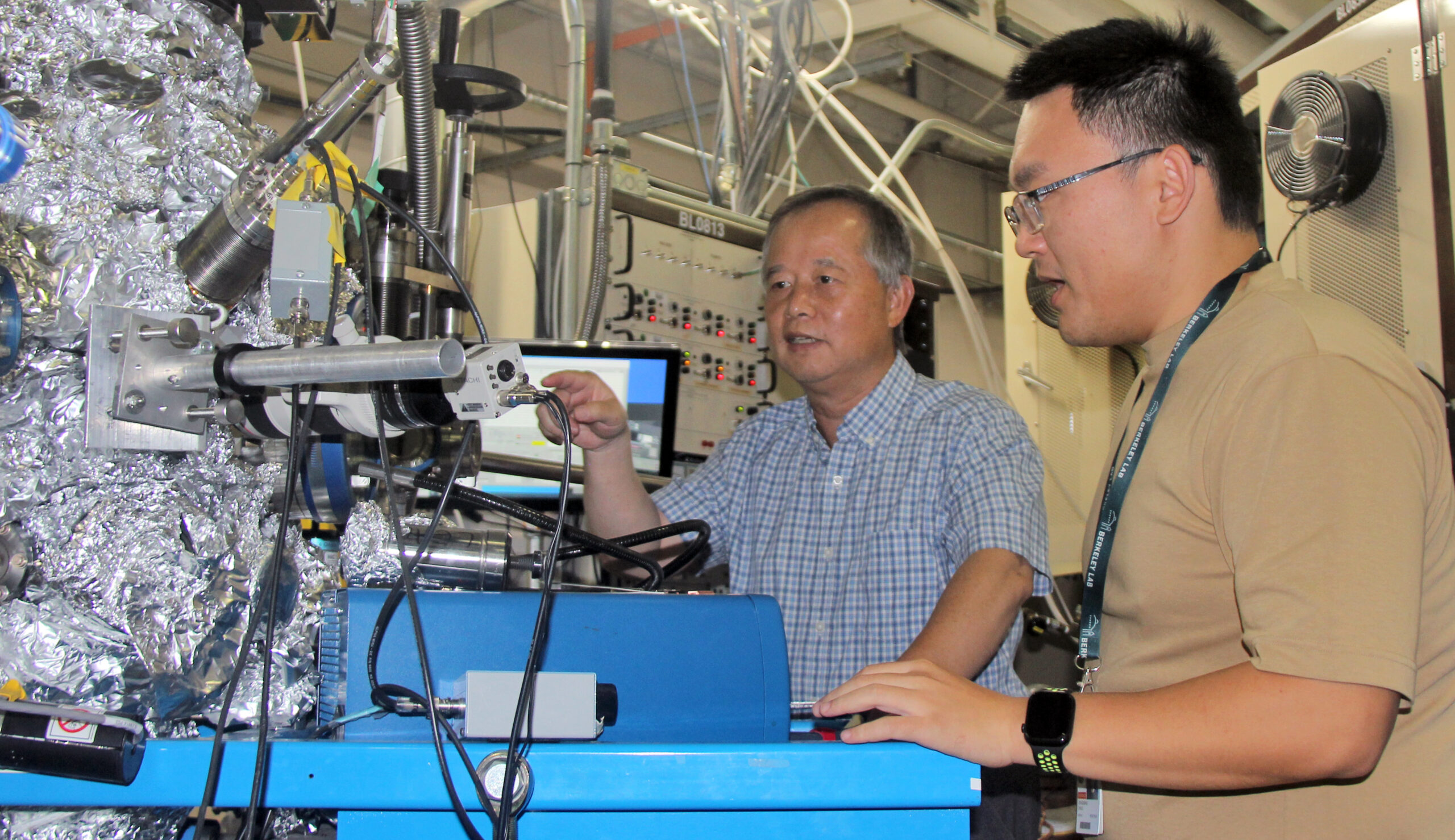
Today, hydrogen is stored primarily as a gas at very high pressures (up to 700 times atmospheric pressure). This is not only expensive, it’s also impractical. But the reason it’s necessary is that, although hydrogen has the highest gravimetric capacity (energy per unit mass) of any fuel, its volumetric capacity (energy per unit volume) is very low.
For example, one kilogram of hydrogen has the same energy density as a gallon of gasoline, but because of hydrogen’s low density, even the largest heavy-duty trucks can only transport about 500 kg of it. Moreover, the large quantities of hydrogen needed for stationary applications such as microgrids (i.e., standalone, localized energy networks) and seasonal energy storage would immediately encounter space limitations.
Condensed-phase materials that reversibly release hydrogen, such as metal hydrides and metal-organic frameworks (MOFs), as well as liquid compounds such as methylcyclohexane (CH3C6H11), are a promising alternative with higher volumetric capacities and much lower rehydrogenation pressures.
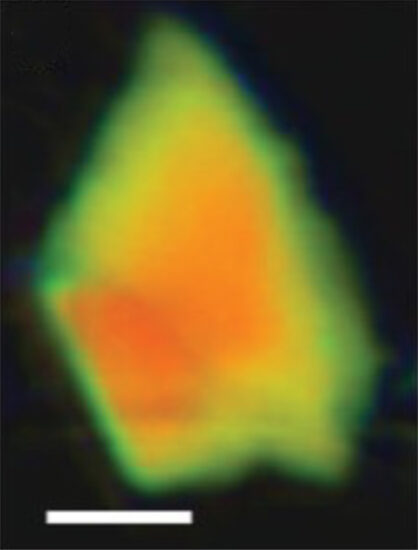
One mystery that ALS tools have helped resolve concerns the rate-limiting step controlling the release of hydrogen from a metal hydride. A common assumption is that the release of H2 through the recombination of hydrogen atoms at a particle’s surface is fast. Therefore, subsurface processes, such as hydrogen diffusion, were expected to control the release rate.
STXM experiments on lithium amide (LiNH2) revealed an inverse core-shell structure with a hydrogen-rich surface and hydrogen-free core. This meant that the rate-limiting step needed to shift from internal hydrogen diffusion to the formation of hydrogen gas at the surface. STXM allowed the hydrogen content and nitrogen-bonding environment to be probed, easily distinguishing Li3N at particle centers from Li2NH at the surfaces.
Operando APXPS experiments involving hydrogen release from sodium aluminum hydride (NaAlH4), a long-studied but incompletely understood storage material, showed that surface hydroxides facilitate hydrogen release, rather than serving as a diffusion barrier.
These results led the HyMARC team to revise its strategies for improving hydrogen desorption rates from metal hydrides. Rather than searching for additives to facilitate subsurface hydrogen diffusion, they now seek approaches such as nanoscaling to destabilize the hydride surface.
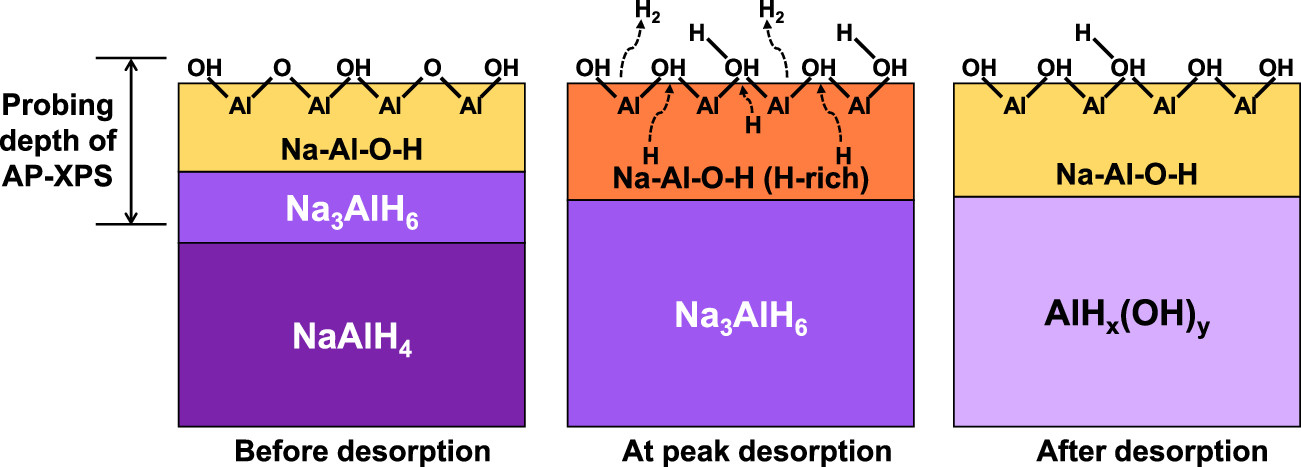
Another transformative discovery concerns the mechanism by which single platinum atoms on cerium oxide (CeO2) dehydrogenate cycloalkanes—single-ring hydrocarbons with single carbon–carbon bonds.
Ji Su, a materials scientist in Berkeley Lab’s Energy Storage and Distributed Research Division, and his coworkers synthesized individual platinum atoms with neighboring oxygen vacancies on cerium oxide (Pt1/CeO2). Dehydrogenation of cycloalkanes using this as a catalyst is more than 300 times faster than conventionally supported platinum nanoparticles. XAS spectra of the Ce M-edge revealed the Ce oxidation state and provided validation for first-principles electronic structure calculations.
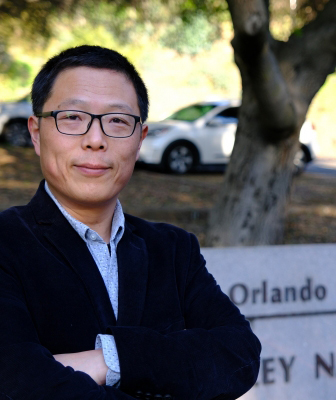
These data support a catalytic mechanism in which Pt1/CeO2 exhibits a “super-synergistic effect” between the platinum atom and its support. Redox coupling between the platinum and cerium ions enables adsorption and activation of large molecules such as cyclohexane with sufficient versatility to drive removal and addition without requiring multiple reaction sites. This shows that the combination of a single platinum atom with cerium ions, coupled with a switchable oxygen coordination environment, can catalyze multiple reactions at a single site.
Moreover, unlike platinum nanoparticles, Pt1/CeO2 is remarkably selective, producing only benzene from cylcohexane at 250 °C. “These discoveries have important economic implications, as minimizing the content of platinum-group metals in catalysts is a key aspect of cost-reduction strategies,” said Su.
HyMARC is now focusing much of its effort on understanding phenomena that emerge when quantities are raised to industrial scales. Access to ALS facilities remains essential. The high photon flux and ability to probe light elements will enable HyMARC researchers to detect small changes in laboratory-scale samples that could become problems when large amounts of storage materials are cycled over long periods of time.
The ambitious goals of the DOE’s Energy Earthshots increase the urgency of finding solutions to the hydrogen storage problem. “ALS resources have become an invaluable part of HyMARC’s toolkit. The recent AP renewal is a tribute to its impact on the field of hydrogen storage,” said Allendorf. “I’m certain the ALS will continue to be an influential player helping us overcome these difficult challenges.”