Scientists have developed a robust and label-free method to probe the chemical underpinnings of developing bacterial biofilms. Almost all bacteria can form biofilms—dynamic communities of cells enclosed in self-produced matrices of polymers that stick to other bacteria or surfaces in water-containing environments. Coordinated collectively, these bacteria defend against antagonists, break down recalcitrant materials, and produce biofuels. Researchers from Berkeley Lab, Lawrence Livermore National Lab, and UC Berkeley coupled infrared (IR) rays from ALS Beamline 1.4 to the first open-channel microfluidic platform to determine the chemistry that shapes biofilm development. This combination of synchrotron radiation–based Fourier transform infrared (SR-FTIR) spectromicroscopy and the microfluidic platform will have a significant impact on several scientific disciplines that require chemical–scale information on biofilm phenotype and function, including Berkeley Lab’s bioenergy efforts and subsurface biogeochemical studies.
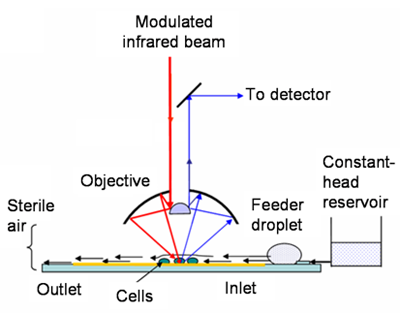
The mid-IR light (~2.5–15.5 mm wavelength, or ~4000–650 cm–1 wavenumber) from Beamline 1.4 is ideal for studying living bacteria individually or in small groups. This light is nondestructive and provides label-free, fingerprint-like spectra originating from the characteristic vibrational frequencies of various functional groups in biomolecules. Fluorescence microscopy yields high-resolution images and measurements of specifically labeled molecules in fixed samples or in engineered or labeled living cells. In contrast, SR-FTIR spectromicroscopy continuously monitors the changing contents in living samples without labeling, detecting functional groups that indicate the types of molecules present and the changes in their chemical composition or conformation. As a result, SR-FTIR spectromicroscopy can be used to study dynamic processes in bacteria living in water as they respond to stimuli, forming and dissolving biofilms.
Because water strongly absorbs mid-IR light, it can completely obscure the IR signals when present even in a thin layer. As a result, bottlenecks in experiments occur when trying to get the optical thickness of water “just right”—enough to support life and ensure the validity of cells in biofilms, but not so much that it masks the molecular fingerprints of interest. To address this problem, the research team fabricated an open-channel microfluidic device that maintains a thin layer of water (10 µm) in a channel that produces minimal IR interference and offers a controllable flow rate.
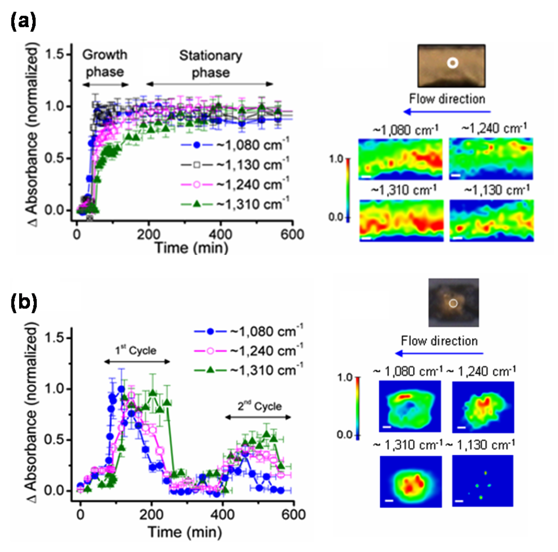
To evaluate this technique’s potential, researchers studied why the antimicrobial agent mitomycin-C (MMC) does not kill some E. coli in biofilms, focusing on the entrance of MMC into bacterial cells and the subsequent chemical changes in the biofilm. SR-FTIR spectroscopic measurements were recorded every 5 minutes over a period of 8 hours, beginning just before the influx of MMC. Analyses at different distances from the MMC source at one end of the channel showed different localized biochemical changes in response to the antimicrobial agent. By combining the open-channel system with SR-FTIR spectromicroscopy, researchers were able to maintain living bacteria on biofilm over a long period of time while capturing continuous measurements and images.
In another study, researchers focused on how environmental conditions influence the initial growth and development of bacteria on surfaces. They compared the spectroscopic changes in microchannels and microwells, which have fast and slow rates of nutrient influx and waste product removal, respectively. Different markers of biofilm formation increased asymptotically in microchannels but increased and decreased cyclically in microwells. One such marker, glycocalyx carbohydrate, was abundant in microchannels at sites with evidence of extensive biofilm growth, confirming the hypothesis that this molecule is crucial to biofilm formation and persistence. Additionally, the microwells’ comparatively lower level of glycocalyx carbohydrate suggests a weaker biofilm attachment.
Researchers demonstrate here the ability of the open-channel microfluidic platform to maintain the functionality of living cells while enabling high-quality SR-FTIR spectromicroscopy measurements. Future applications of the SR-FTIR–based microfluidics approach may help explain why some bacteria maintain biofilms in given environments while others do not.
Contact: Hoi-Ying Holman
Research conducted by: H-Y. Holman, Z. Hano, and E. Wozel (Berkeley Lab); R. Miles (Lawrence Livermore National Lab); and L.M. Anderson and H. Yang (University of California, Berkeley).
Research funding: U.S. Department of Energy (DOE), Office of Biological & Environmental Research. Operation of the ALS is supported by DOE, Office of Basic Energy Sciences.
Publication about this research: H-Y. Holman, R. Miles, Z. Hao, E. Wozel, L.M. Anderson, and H. Yang, “Real-time chemical imaging of bacterial activity in biofilms using open-channel microfluidics and synchrotron FTIR spectromicroscopy,” Anal. Chem. 81, 8564 (2009).
ALS SCIENCE HIGHLIGHT #215