Future technology will require energy storage systems that have much larger storage capability, rapid charge/discharge cycling, and improved endurance. Progress in these areas demands a more complete understanding of the processes involved in energy storage, from the atomic scale to the device level. Now, using soft x-ray absorption spectroscopy (XAS) under operating conditions (“in operando”), researchers have found that the interfacial electric fields generated during charging of the electrode/electrolyte interface of a supercapacitor can induce changes in the structure and bonding of its graphitic carbon electrodes. The research could lead to better, more reliable strategies for improving the capacity and efficiency of electrical energy storage systems needed to meet the burgeoning demands of consumer, industrial, and green technologies.
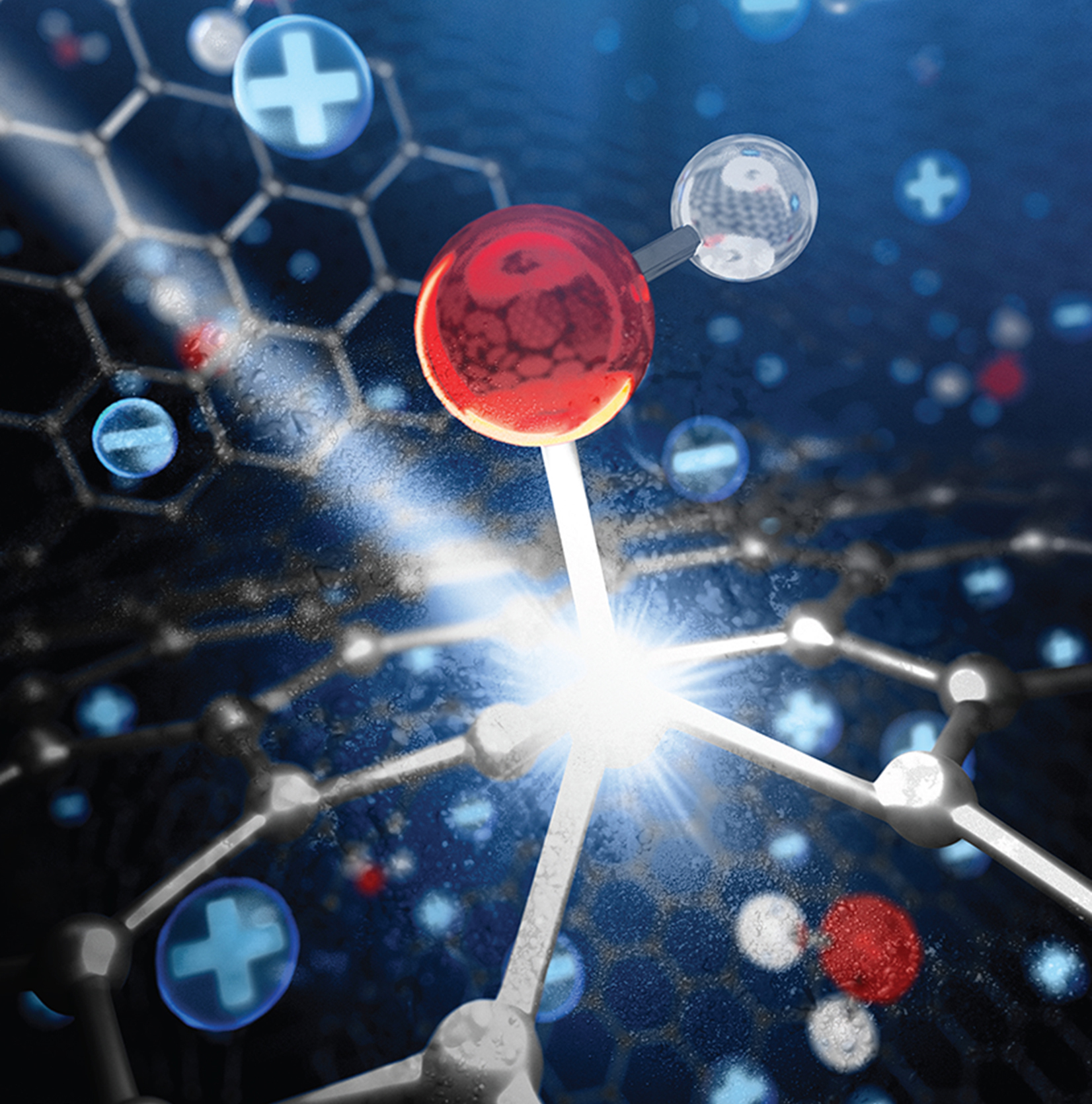
Electrode/electrolyte interfaces are critical to all electrochemical energy storage technologies, including supercapacitors, yet we still don’t fully understand how the physiochemical properties of these devices are altered by the interfacial electric field generated during charging. While the structural and dynamical responses of the electrolyte to an applied potential have been extensively studied, the analogous responses of the electrode material during operation remain largely unexplored, even for widely used materials such as graphitic electrodes. The electronic structure of the electrode is conventionally considered to be static, with charge accumulation or depletion as the only response to polarization of the interface. This lack of understanding of the dynamic physiochemical changes of the electrode is largely due to the paucity of experimental and theoretical methods for characterization of the electrode electronic structure during operation.
A team led by researchers from Lawrence Livermore National Laboratory (LLNL) has developed an in operando XAS capability at the ALS that is tightly coupled with theoretical modeling efforts at LLNL and at Berkeley Lab’s Molecular Foundry. For this work, the team used its recently developed three-dimensional nanographene (3D-NG) bulk electrode material as a model graphitic electrode. Graphitic supercapacitors are ideal model systems to probe interfacial phenomena because they are chemically relatively stable, extensively characterized experimentally and theoretically, and are highly relevant technologically.
At ALS Beamlines 7.0.2 and 8.0.1, the researchers analyzed the working electrode of a functioning supercapacitor in an aqueous NaCl electrolyte using carbon K-edge XAS, which selectively probes the electrode/electrolyte interface. The data recorded under positive electrical bias revealed two new resonances. As the applied positive bias changed, the intensities of these resonances changed systematically but independently of each other, suggesting at least two different physiochemical effects at play. The resonances also dissipated upon removal of the applied bias, indicating that the associated processes are largely reversible. Ab initio simulations of XAS spectra under bias were employed to help identify specific charge-induced atomic-level and mesoscale phenomena that can explain the observed bias-dependent resonances.
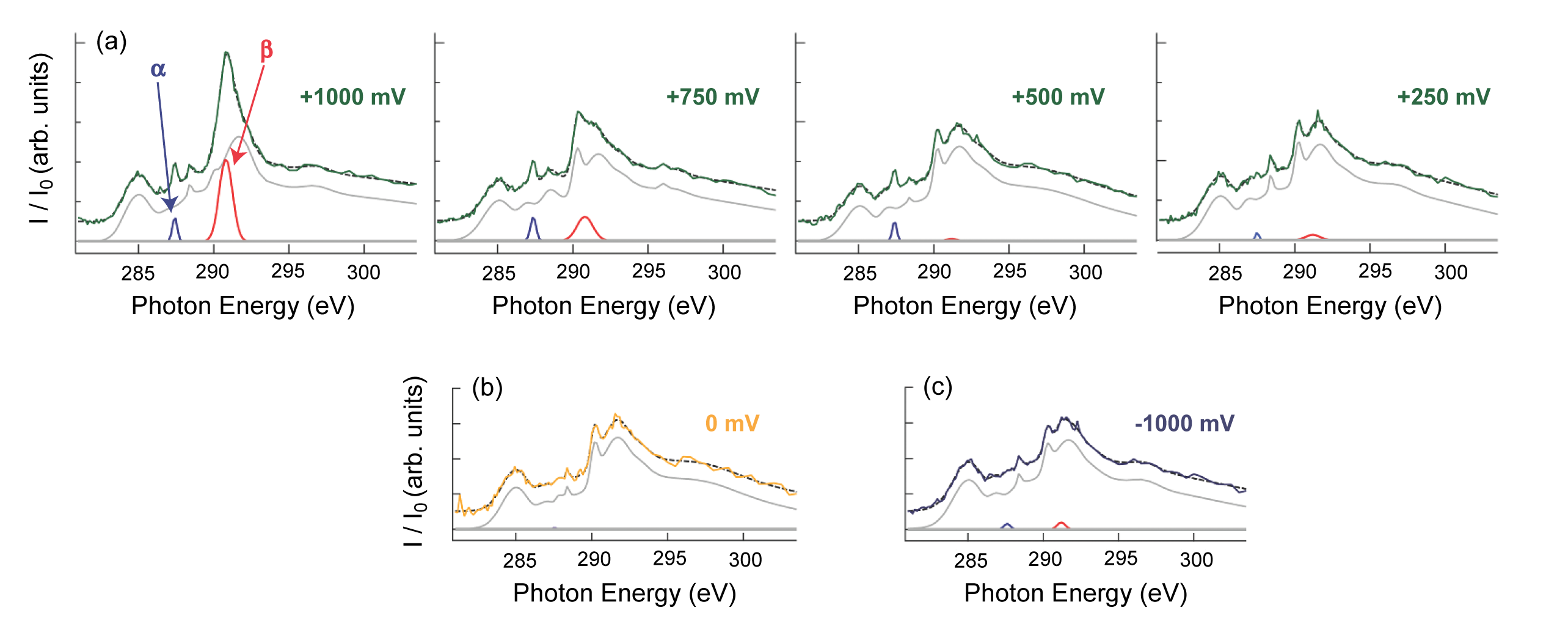
The appearance of the first of the two new peaks under positive bias is consistent with the specific adsorption of anions on the electrode surface, most likely OH–. The charge transfer that accompanies covalent adsorption of OH– onto the electrode generates a pseudocapacitive channel for electrical energy storage. The energy and evolution of the second new peak suggest that strain-induced morphological changes are induced in the 3D-NG electrode during charging. From simulations of the spectral responses of graphene sheets to compressive and tensile stress, the researchers concluded that charging occurs unevenly in the hierarchical pore structure of 3D-NG, and that the second peak arises from local flattening of the curved graphene structures—a uniquely mesoscale response, in contrast to the atomic-level response represented by the first peak.
Discovering that the electronic structure of graphitic carbon supercapacitor electrodes can be tailored by charge-induced electrode/electrolyte interactions opens a new window toward more efficient electrochemical energy storage systems. In addition, the experimental and modeling techniques developed during the research are readily applicable to other energy storage materials and technologies.
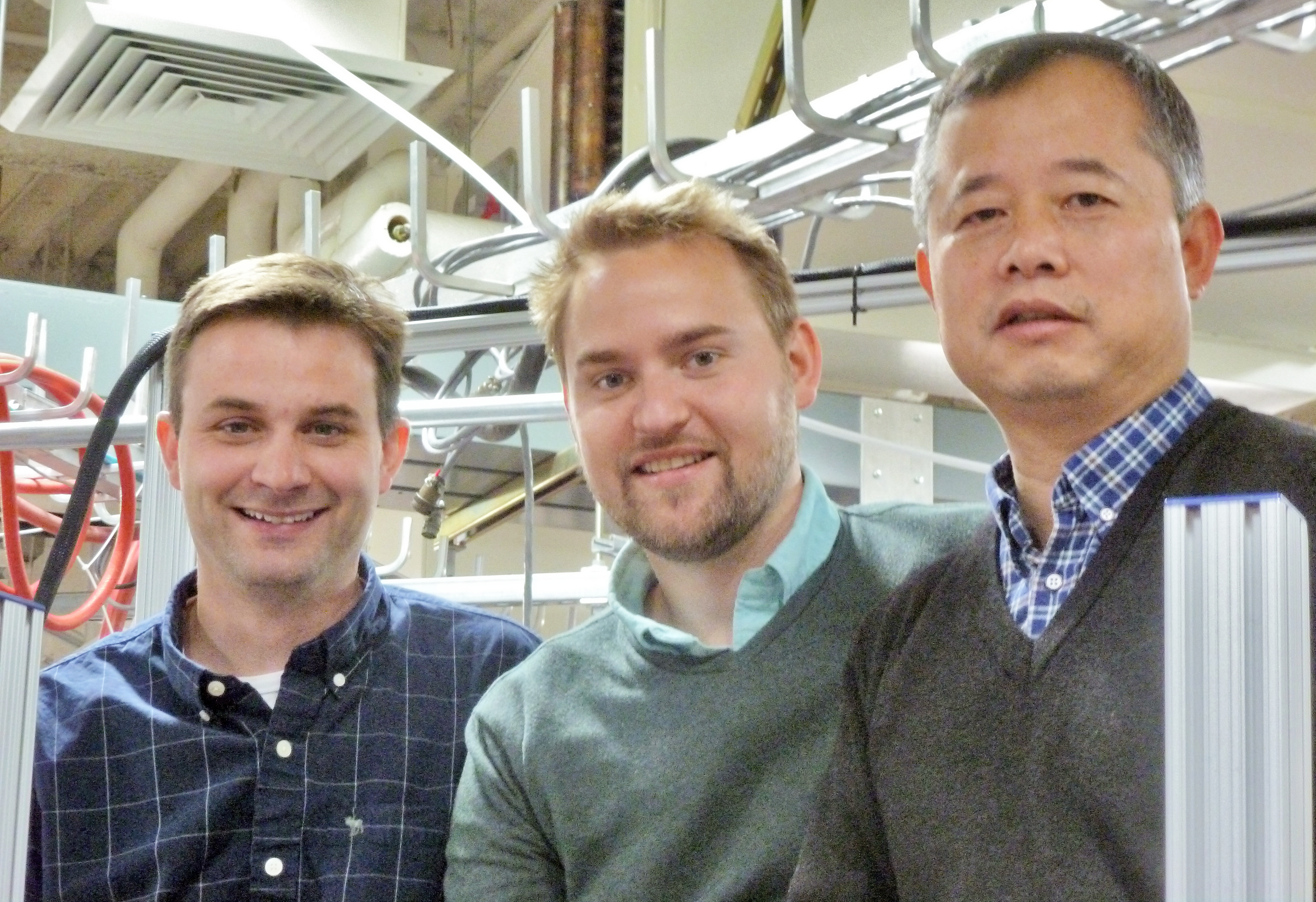
Contacts: Jonathan Lee and Michael Bagge-Hansen
Research conducted by: M. Bagge-Hansen, B.C. Wood, T. Ogitsu, T.M. Willey, I.C. Tran, A. Wittstock, M.M. Biener, M.D. Merrill, M.A. Worsley, T.F. Baumann, T. van Buuren, J. Biener, and J.R.I. Lee (Lawrence Livermore National Laboratory); M. Otani (National Institute of Advanced Industrial Science and Technology, Japan); D. Prendergast (Lawrence Berkeley National Laboratory); and J.-H. Guo and C.-H. Chuang (ALS).
Research funding: Lawrence Livermore National Laboratory, Japan–U.S. Cooperation Project for Research and Standardization of Clean Energy Technologies. Operation of the ALS is supported by the U.S. Department of Energy, Office of Basic Energy Sciences.
Publication about this research: M. Bagge-Hansen, B.C. Wood, T. Ogitsu, T.M. Willey, I.C. Tran, A. Wittstock, M.M. Biener, M.D. Merrill, M.A. Worsley, M. Otani, C.-H. Chuang, D. Prendergast, J. Guo, T.F. Baumann, T. van Buuren, J. Biener, and J.R.I. Lee, “Potential-Induced Electronic Structure Changes in Supercapacitor Electrodes Observed by In Operando Soft X-Ray Spectroscopy,” Adv. Mater. 27, 1512 (2015).
ALS SCIENCE HIGHLIGHT #313