Solid-oxide fuel cells (SOFCs) are a promising path toward the “clean” conversion of chemical energy to electrical energy with little or no carbon dioxide emission. With the help of the ALS, researchers from MIT recently found a way to treat SOFC cathode surfaces so that they perform better and last longer. Ambient-pressure x-ray photoelectron spectroscopy (AP-XPS) and ambient-pressure x-ray absorption spectroscopy (AP-XAS) were critical to the researchers’ success in uncovering the physical principles behind this improvement, which can reduce energy losses in fuel cells by half, making them twice as efficient and less costly.
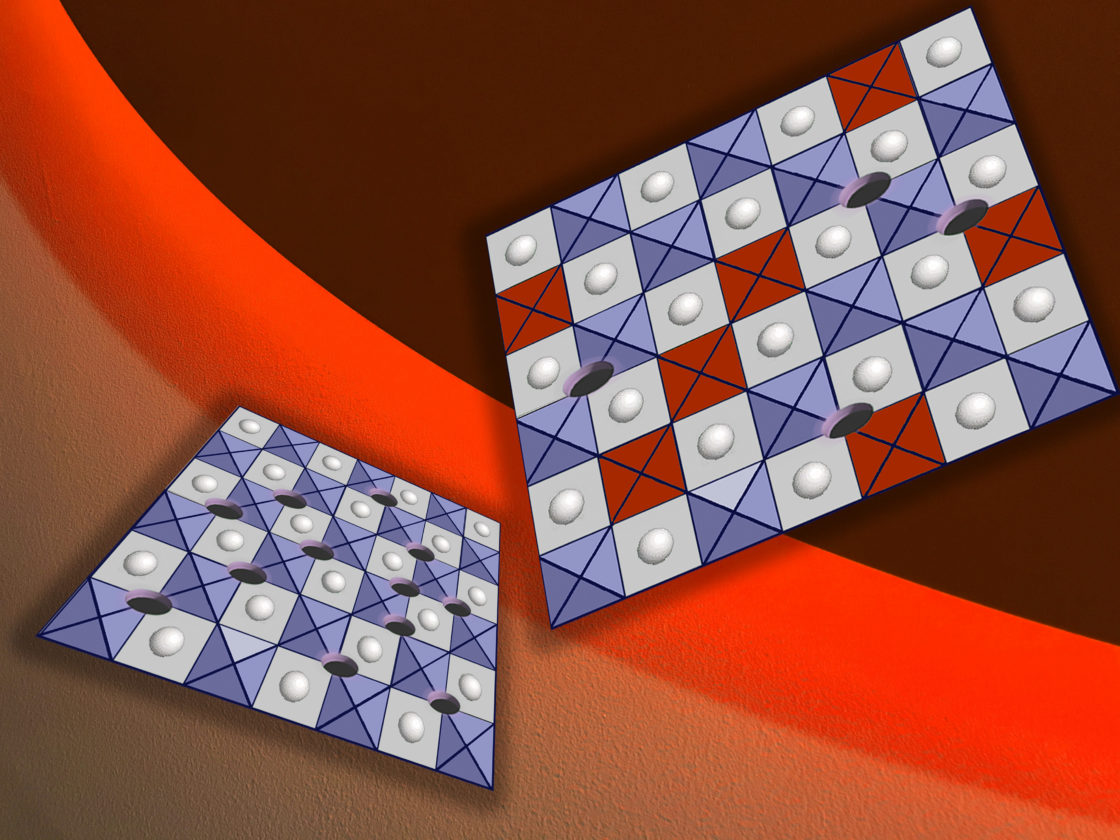
SOFCs are devices that convert the chemical energy in fuels (hydrogen, syngas, natural gas, etc.) directly into electricity, with very high efficiency and zero exhausted pollutants. However, one limiting factor preventing the wide deployment of this clean energy-conversion technology is the degradation of the cathode, usually made of a perovskite oxide, under operating conditions of high temperature (greater than 500 °C) in an oxidizing atmosphere (air). Earlier work had found that an important reason for this degradation is the segregation and phase separation of dopants (i.e., strontium), deposited on the cathode surface to catalyze fuel-cell reactions. The segregated strontium further turns into secondary surface phases such as SrO, which blocks the surface electrocatalytic activity. The SOFC community has been working for years to find a workable solution to this problem.
In a previous study, the researchers pinpointed two physical driving forces for this strontium surface segregation: electrostatic interactions and the minimization of elastic energy. In this work, the group focused on electrostatic interactions at the surface of La0.8Sr0.2CoO3 (LSC), a state-of-the-art perovskite cathode material with high electrochemical performance. To reduce the concentration of oxygen vacancies, which attract strontium toward the surface through electrostatic attraction, the researchers deposited binary oxides (HfO2, TiO2, ZrO2, and V2O5) on the surface at submonolayer coverages, using a feasible chemical bath method. They found that HfO2 and TiO2 greatly enhanced the surface stability of LSC against strontium segregation and improved performance by a factor of up to 30.
To test whether they were indeed tuning the surface oxygen vacancy content, the researchers performed AP-XPS and AP-XAS experiments at ALS Beamline 9.3.2. AP-XPS is a novel and powerful materials-characterization tool that can only be found in a few synchrotron beamlines around the world. Traditionally, x-ray photoelectron spectroscopy can only be performed under ultrahigh-vacuum conditions, far from realistic operating conditions for fuel-cell cathode materials. Ambient-pressure studies at the ALS allow the observation of changes in the chemical composition of LSC surfaces in environments close to the operating conditions of SOFC cathodes, providing valuable, meaningful, and operationally relevant results.
At high temperature (550 °C) and high oxygen pressure (0.76 Torr), the AP-XPS data showed that the LSC-Hf sample exhibited the least amount of surface-segregated strontium. Co L-edge and O K-edge XAS as well as valence-band spectra showed that the addition of Hf reduced the concentration of surface oxygen vacancies (missing oxygen sites). This effect largely mitigates the electrostatic driving force of strontium surface segregation, thus greatly improving the stability of LSC surfaces. The results demonstrate that, in an ideal cathode material, a balance must be struck between reactivity and stability, challenging the conventional wisdom that oxygen-vacancy concentration has a solely beneficial effect on electrochemical performance.
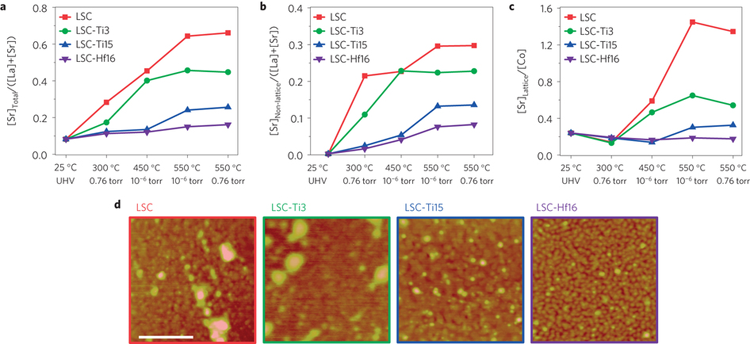
The researchers note that this approach is not material specific; they are currently working to apply it to other perovskite oxide systems. Also, strontium surface segregation is not just an issue for SOFC cathode materials. Other applications, such as thermochemical fuel production, solid-oxide electrolysis cells, and oxygen gas-separation membranes, all suffer from the same issue, albeit under different gas atmospheres. Fundamentally, the aim is to resolve the atomic structure formed on these chemically modified surfaces—something that will need more synchrotron work to accomplish.
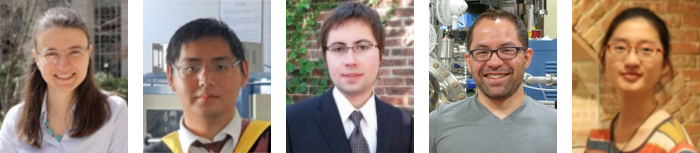
Contact: Bilge Yildiz
Research conducted by: N. Tsvetkov, Q. Lu, L. Sun, and B. Yildiz (Massachusetts Institute of Technology) and E. Crumlin (ALS).
Research funding: National Science Foundation and National Aeronautics and Space Administration. Operation of the ALS is supported by the US Department of Energy, Office of Basic Energy Sciences.
Publication about this research: N. Tsvetkov, Q. Lu, L. Sun, E.J. Crumlin, and B. Yildiz, “Improved chemical and electrochemical stability of perovskite oxides with less reducible cations at the surface,” Nature Materials 15, 1010 (2016). doi:10.1038/nmat4659
ALS SCIENCE HIGHLIGHT #339