In a first-of-its-kind experiment, ALS researchers demonstrated a new, direct way to study the inner workings of a phenomenon in chemistry known as an “electrochemical double layer” that forms where liquids meet solids—where battery fluid meets an electrode, for example. Scientists can now directly probe this previously elusive layer of chemistry thanks to a unique x-ray toolkit developed at the ALS. These x-ray tools and techniques could be extended to provide new insight into battery performance and corrosion, a wide range of chemical reactions, and even biological and environmental processes that rely on similar chemistry.
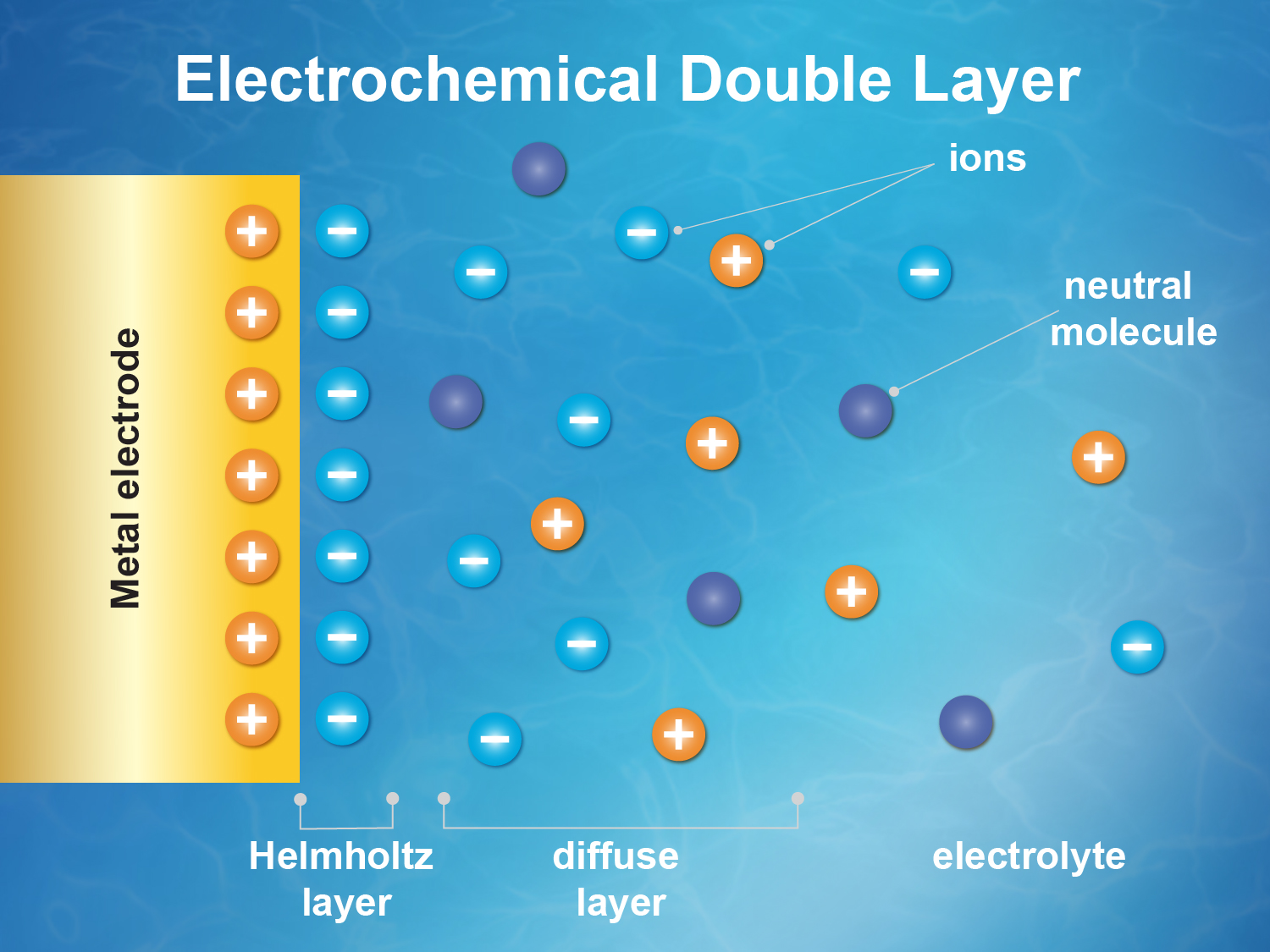
The problem of understanding electrified solid/liquid interfaces has been known for many years, but researchers trying to explain what’s at work have mostly been limited to running computer simulations and modeling. In a battery, for example, the electrochemical double layer describes the layer of charged atoms or molecules in the battery’s fluid that are drawn in and cling to the surface of the electrode because of their opposite electrical charge—an essential step in battery operation—and a second and closely related zone of chemical activity that is affected by the chemistry at the electrode’s surface. The complex molecular-scale dance of charge flow and transfer within a battery’s double layer is central to its function, and the ability to directly probe any attribute of the double layer would be a significant advancement.
A team of researchers from Berkeley Lab and Shanghai have now demonstrated the ability to measure changes in the electric potential within the double layer under operating conditions. Essentially, they can now create a direct map, independent of a model, showing how the potential within the double layer changes with respect to other key parameters.
An important breakthrough enabling this experiment was the ability to combine ambient-pressure x-ray photoelectron spectroscopy (APXPS) at ALS Beamline 9.3.1 with “tender” x-rays, which are intermediate in energy between soft and hard x-rays. Tender x-rays provide the right penetrating power to the escaping photoelectrons, allowing measurement of the electric potential profile for water and pyrazine molecules across the solid/liquid interface in response to changes in the electrode potential and the electrolyte concentration.
To do this, the researchers used a gold electrode and an aqueous electrolyte containing a neutral spectator molecule called pyrazine (C4H4N2), introduced as a uniformly distributed, independent molecular probe. In addition to being element- and chemical-specific, XPS provides information about the local potentials of atoms that undergo photoionization. By measuring the shifts in core-level binding energies of the elements in the liquid layer (such as N 1s from pyrazine and O 1s from water) as a function of the applied potential at the electrode, it is possible to determine local potentials within the liquid layer. Moreover, the width of the core-level peaks broaden as the potential in the double layer increases, providing a key spectroscopic parameter to directly measure double-layer properties.
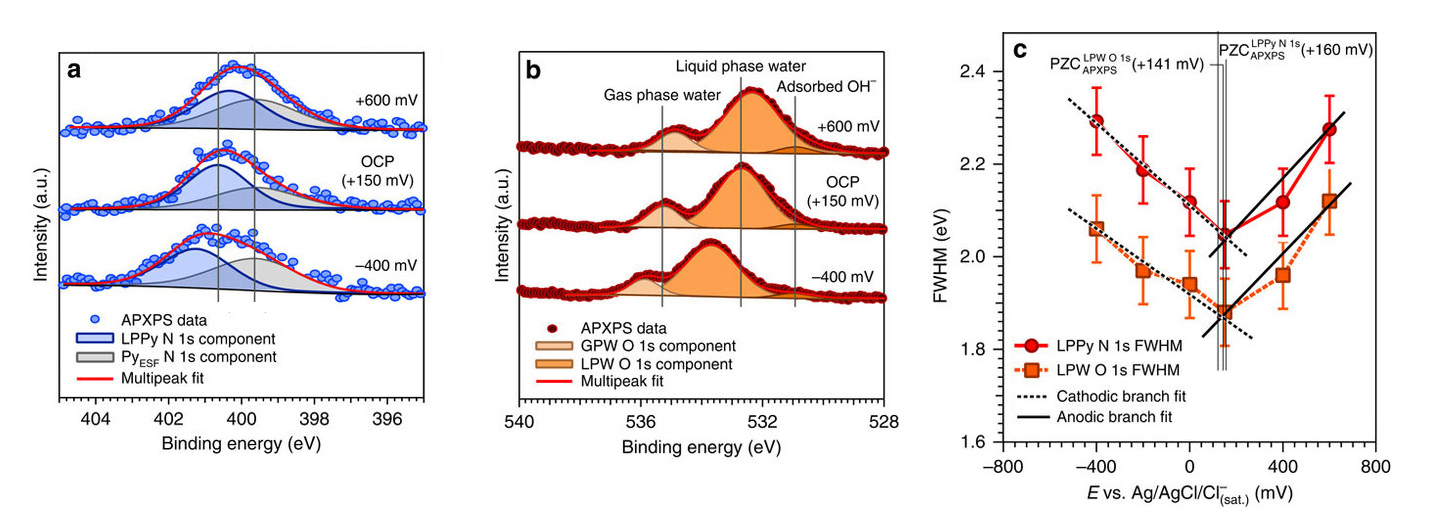
The data provided a precise measure of the drop in the electric potential within the double layer. The measurements also enabled determination of the potential of zero charge (PZC), the value of applied potential at which no net charge density is present at the electrode surface. These results help narrow the list of candidate models explaining the double layer. Importantly, the technique is well suited to active chemistry, and there are plans to add new capabilities to make this technique more robust for studying finer details during the course of (electro)chemical reactions and to bring in other complementary x-ray techniques.
Solid/liquid interfaces are key for all kinds of research, from batteries and fuel cells to artificial photosynthesis. The information gained by applying these new tools will provide guidance for theoretical models as well as materials design and the development of improved electrochemical, environmental, biological, and chemical systems.
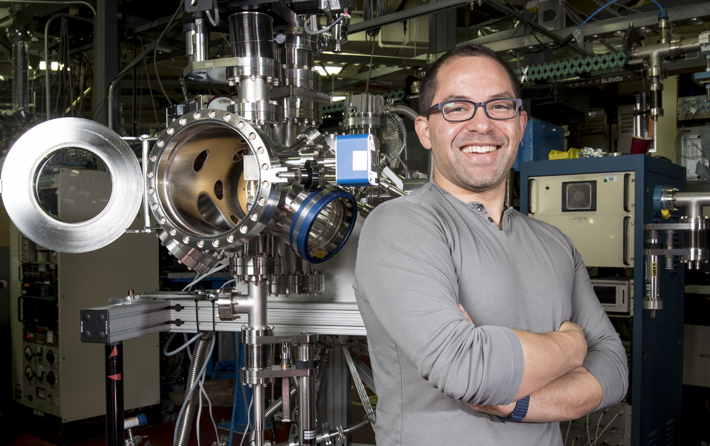
Contact: Ethan Crumlin
Research conducted by: M. Favaro [ALS, Joint Center for Artificial Photosynthesis (JCAP), and Berkeley Lab], B. Jeong (ALS and Gwangju Institute of Science and Technology, Republic of Korea), P.N. Ross (Berkeley Lab), J. Yano (JCAP and Berkeley Lab), Z. Hussain (ALS), Z. Liu (ALS, Shanghai Institute of Microsystem and Information Technology, and ShanghaiTech University), and E.J. Crumlin [ALS and Joint Center for Energy Storage Research (JCESR)].
Research funding: U.S. Department of Energy, Office of Basic Energy Sciences (DOE BES); National Natural Science Foundation of China; and the Chinese Academy of Sciences–Shanghai Science Research Center. Operation of the ALS is supported by the DOE BES.
Publication about this research: M. Favaro, B. Jeong, P.N. Ross, J. Yano, Z. Hussain, Z. Liu, and E.J. Crumlin, “Unravelling the Electrochemical Double Layer by Direct Probing of the Solid/Liquid Interface,” Nat. Commun. 7, 12695 (2016). doi:10.1038/ncomms12695
ALS SCIENCE HIGHLIGHT #340