SCIENTIFIC ACHIEVEMENT
At the Advanced Light Source (ALS), a time-resolved study using infrared spectromicroscopy in a carefully controlled environment revealed why enzymes get bogged down when trying to break down cellulose from plants.
SIGNIFICANCE AND IMPACT
The work sheds new light on the challenge of extracting the sugars locked up in plants for use in making petroleum-free fuels, chemicals, and medicines.
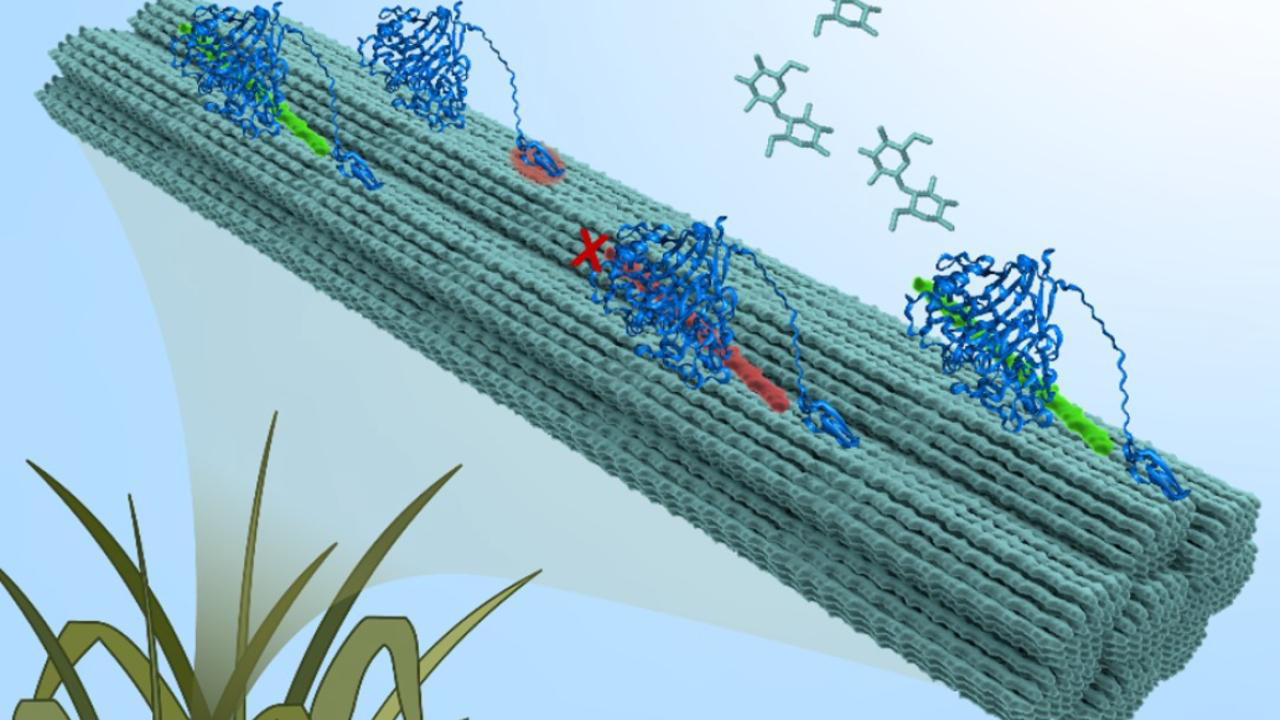
Cellulose: a sweet deal
Using microbes to convert grasses, weeds, wood, and other plant residues into sustainable products will be key to achieving carbon neutrality and could even help eliminate drug shortages. But cellulose, the tough tissue that makes up a large proportion of herbaceous and woody plant bodies, is hard to break down into its composite sugars, which the microbes need to build other molecules. Only organisms that have evolved specialized enzymes, or those that host microbiomes of those organisms, are able to get sugars from cellulose-rich plant matter.
In this work, researchers combined a novel microfluidic device with infrared spectromicroscopy to study how a cellulose-degrading enzyme works in real time, with the ultimate goal of developing more-efficient methods to convert plant waste into sweet ingredients. These sugars are key to establishing a bioeconomy built on cycling renewable carbon for biofuel, biochemical, and biomaterial alternatives to fossil fuel–sourced versions.
A novel “open” microfluidic device
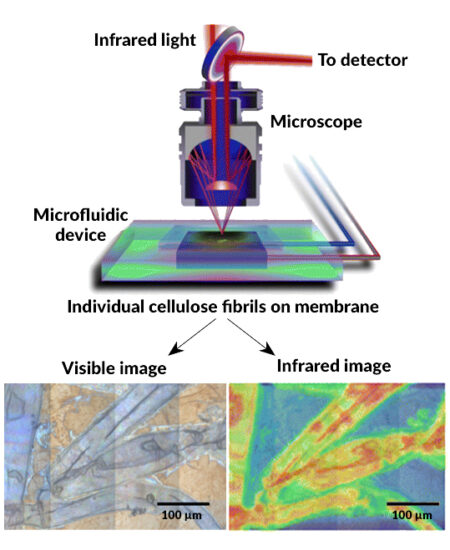
Until now, the enzymatic breakdown (hydrolysis) of cellulose was a challenging biochemical process to study, in part due to limitations in researchers’ ability to carefully control, and observe, the sample environment during the depolymerization of cellulose. To overcome these limitations, the researchers designed an experimental system that can provide information about how the atomic structure of the cellulose changes while the enzyme is working. The system consists of a small disk-like device that holds a tiny quantity of fluid containing cellulose from green algae and a tiny amount of an enzyme derived from fungi. When the device moves the two fluids together, the reaction can begin. Importantly, the device is partially open rather than sealed shut, enabling on-demand access to the sample while maintaining the necessary conditions for the enzymatic reaction and data collection.
At the ALS, the device was placed in the path of infrared light from Beamline 1.4, where the researchers performed Fourier-transform infrared spectroscopy. The exceptional brightness of the infrared beam allowed for precise, real-time snapshots of the vibrational modes of molecules of interest in the sample. Changes in spectral features (location, shape, and intensity of peaks) can be used to track changes in cellulose abundance, molecular ordering, and chemical bonds or bond environments.
Hydrogen bonds get in the way
Cellulose is composed of many glucose molecules, each joined together by a single covalent bond. The long chains of glucose are twisted together into complex rope-like structures called fibrils, which stay in that formation thanks to many hydrogen bonds between the tightly organized glucoses. Analysis of the infrared data revealed spatial heterogeneity in the kinetics of cellulose hydrolysis and indicated that the hydrogen bonds in the fibrils are acting as roadblocks for the enzymes, preventing access to the covalent bonds.
The results serve as a proof of concept for the technique, with the potential to enable a new generation of scientific discoveries—especially for the study of physical and chemical properties of biological systems as they occur in their native environments and in real time. The researchers now plan to explore techniques that will help enzymes break through the hydrogen bonds more quickly, to improve efficiency and lower costs of sustainable biomanufacturing.
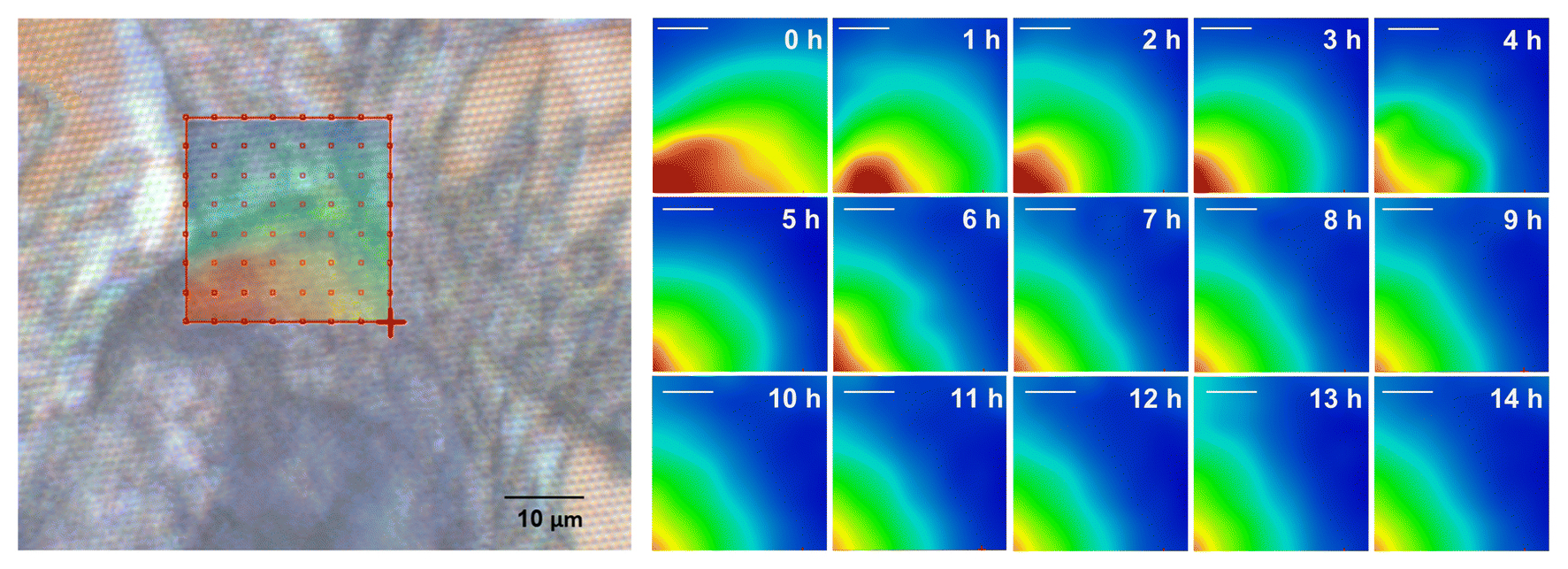
Contact: Hoi-Ying Holman
Researchers: T. Jeoh and J. Danger Nill (UC Davis), and W. Zhao, S.R. Narayanasamy, L. Chen, and H.Y.N. Holman (Berkeley Lab).
Funding: US Department of Energy (DOE), Office of Science, Biological and Environmental Research program. Operation of the ALS is supported by DOE Office of Science, Basic Energy Sciences program.
Publication: T. Jeoh, J. Danger Nill, W. Zhao, S.R. Narayanasamy, L. Chen, and H.Y.N. Holman, “Spatiotemporal dynamics of cellulose during enzymatic hydrolysis studied by infrared spectromicroscopy,” Green Chem. 26, 396 (2023), doi:10.1039/D3GC03279E.
Adapted from the Berkeley Lab press release, “Watching the Enzymes that Convert Plant Fiber into Simple Sugars,” and articles by Elizabeth Boatman and Emily C. Dooley.
ALS SCIENCE HIGHLIGHT #499