SCIENTIFIC ACHIEVEMENT
At the Advanced Light Source (ALS), researchers discovered how a layer of organic molecules on a nanoparticle surface detaches to create a highly catalytic pocket for reducing CO2 to CO.
SIGNIFICANCE AND IMPACT
The ability to probe molecular-scale events under realistic conditions with nanometer resolution will help guide the design of responsive systems for a wide range of applications, from medicine to optoelectronics.

Enzyme-inspired catalysts
In living things, enzymes catalyze chemical reactions with exquisite efficiency and specificity. These enzymes are proteins with amino-acid side chains that optimize the active site for specific reactions. Inspired by nature, scientists have been working to improve electrocatalysts using a similar strategy.
Several years ago, a group led by Peidong Yang at Berkeley Lab invented a system in which chains of organic molecules (ligands) are attached to the surface of a silver nanoparticle. Upon application of an external negative potential, the ligands reversibly dissociate from the nanoparticle. The resulting reaction pocket, called a Nanoparticle/Ordered-Ligand Interlayer (NOLI), supports the markedly efficient conversion of CO2 to CO with 100% selectivity. However, understanding of the mechanism by which electrochemical bias modulates nanoparticle–ligand interactions has been elusive.
Spectroscopic fingerprints
To investigate how ligands on the nanoparticle surface respond to electrochemical conditions and how these responses enhance catalytic performance, the researchers turned to two forms of infrared spectroscopy: synchrotron-based Fourier-transform infrared nanospectroscopy (nano-FTIR) and laser-based surface-enhanced Raman spectroscopy (SERS). The work was also supported by electron microscopy at the Molecular Foundry and density functional theory (DFT) calculations performed at NERSC. The experiment samples consisted of silver (Ag) nanoparticles capped by tetradecylphosphonic acid (TDPA) ligands.
The nano-FTIR was performed at ALS Beamlines 2.4 and 5.4. Both beamlines provide a low-noise, broadband, high-coherence infrared beam, which can be used to detect the characteristic vibrational modes of the ligand while minimizing radiation damage. Furthermore, because of the high spatial resolution and surface sensitivity of nano-FTIR, ligand behavior that occurs only on the nanoparticle surface can be tracked, with a spatial resolution on the order of the nanoparticle size. In addition, the special polarization of this technique (z-polarized) can assist in determining the ligand binding configuration on the nanoparticle surface. Finally, both the beamlines can accommodate a customized liquid cell, which enables the detection of ligand behavior under electrochemical conditions.
SERS was used to follow the evolution of the molecular structure within the NOLI up to more negative bias compared to nano-FTIR. This enables tracking the changes in ligand species through the onset of full detachment. However, SERS is not polarized and hence there is no preferential orientation axis as in nano-FTIR.
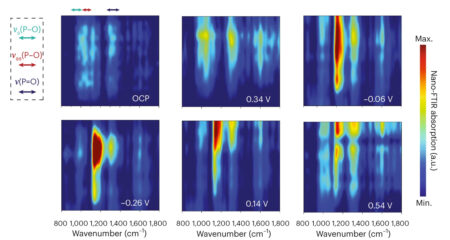
Step-by-step detachment
Analysis of the data revealed that the interlayer pocket forms through a consecutive bond-cleavage mechanism. Initially, the TDPA binds to the Ag surface through two Ag–O bonds (a bidentate binding configuration). Increasing the electrochemical bias triggers a transformation to a monodentate configuration. A second bond cleavage is observed by SERS at higher overpotentials. DFT calculations revealed that each cleavage step was stabilized by water molecules intercalating into the gap, culminating in an ideal microenvironment for CO2 reduction reactions.
The high spatial resolution of the experiments uniquely allows a direct comparison of spectra originating from nanoparticles of varying sizes and evolving statuses, which leads to the conclusion that the bidentate-to-monodentate transformation occurs universally across all nanoparticle surfaces, maintaining consistent ligand structural dynamics irrespective of nanoparticle sizes.
This methodology could be applied to investigate the dynamic response of various interfacial species to multiple external stimuli with nanometer spatial resolution, thus providing fundamental guiding principles for the rational design of responsive ligand–nanoparticle systems with precise dosage, spatial, and temporal control for energy, medical, and optoelectronics applications.
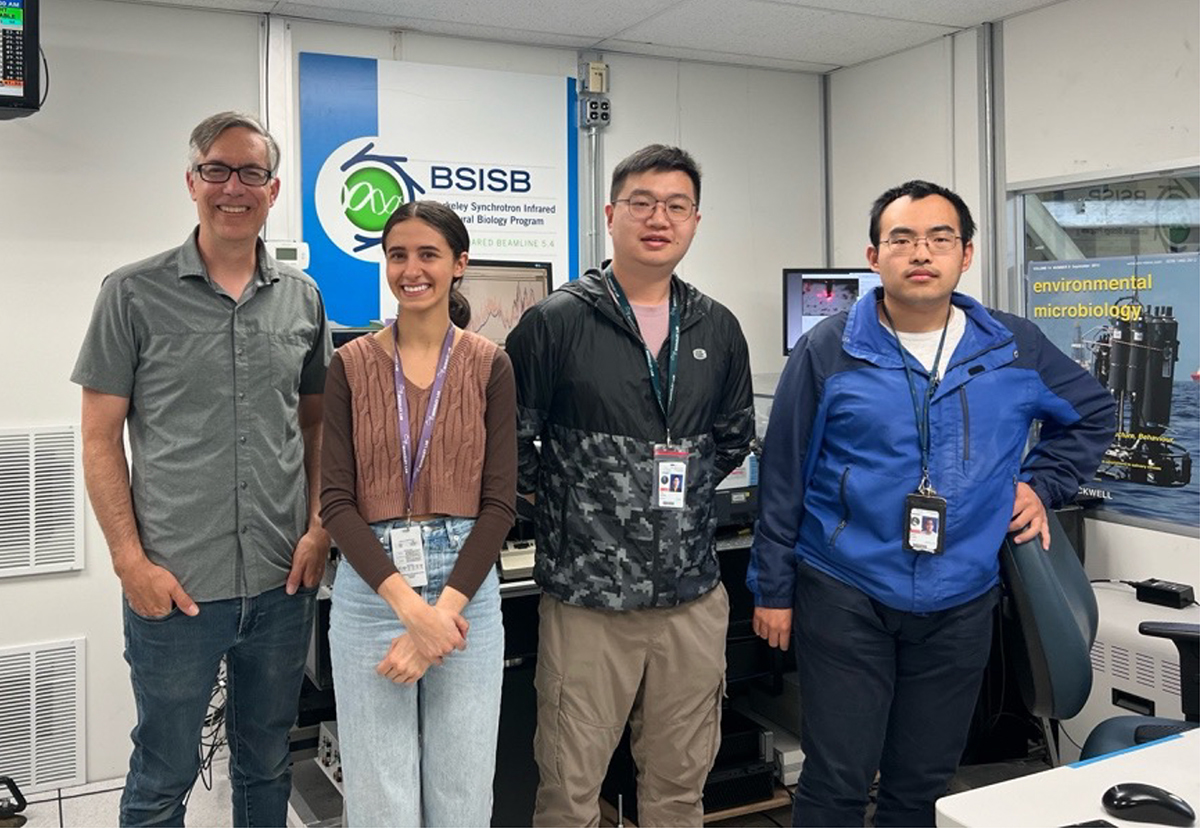
Contacts: Yu Shan and Peidong Yang
Researchers: Y. Shan, X. Zhao, M. Fonseca Guzman, S. Chen, S. Yu, I. Roh, and M.B. Salmeron (UC Berkeley and Berkeley Lab); A. Jana (Berkeley Lab and ALS); K.C. Ng, H. Chen, V. Altoe, and J. Qian (Berkeley Lab); S. Gilbert Corder and H.A. Bechtel (ALS); and P. Yang (UC Berkeley, Berkeley Lab, and Kavli Energy NanoScience Institute.
Funding: US Department of Energy, Office of Science, Basic Energy Sciences program (DOE BES); University of Chinese Academy of Sciences; National Science Foundation; and US-Israel Binational Science Foundation. This research was also supported by Berkeley Lab Laboratory Directed Research and Development program and DOE BES Early Career awards to J. Qian. Operation of the ALS, Molecular Foundry, and NERSC is supported by DOE BES.
Publication: Y. Shan, X. Zhao, M. Fonseca Guzman, A. Jana, S. Chen, S. Yu, K.C. Ng, I. Roh, H. Chen, V. Altoe, S. Gilbert Corder, H.A. Bechtel, J. Qian, M.B. Salmeron, and P. Yang, “Nanometer-resolved observation of electrochemical microenvironment formation at the nanoparticle-ligand interface,” Nat. Catal. 7, 422 (2024), doi:10.1038/s41929-024-01119-2.
ALS SCIENCE HIGHLIGHT #503