SCIENTIFIC ACHIEVEMENT
Advanced Light Source (ALS) studies showed that adding “bulky” organic molecules earlier in solar-film synthesis slows crystal growth, leading to the formation of a protective surface layer that improves durability and efficiency.
SIGNIFICANCE AND IMPACT
These next-gen materials could revolutionize solar-cell technology, offering increased efficiency, lower cost, lighter weight, and flexible solar modules.
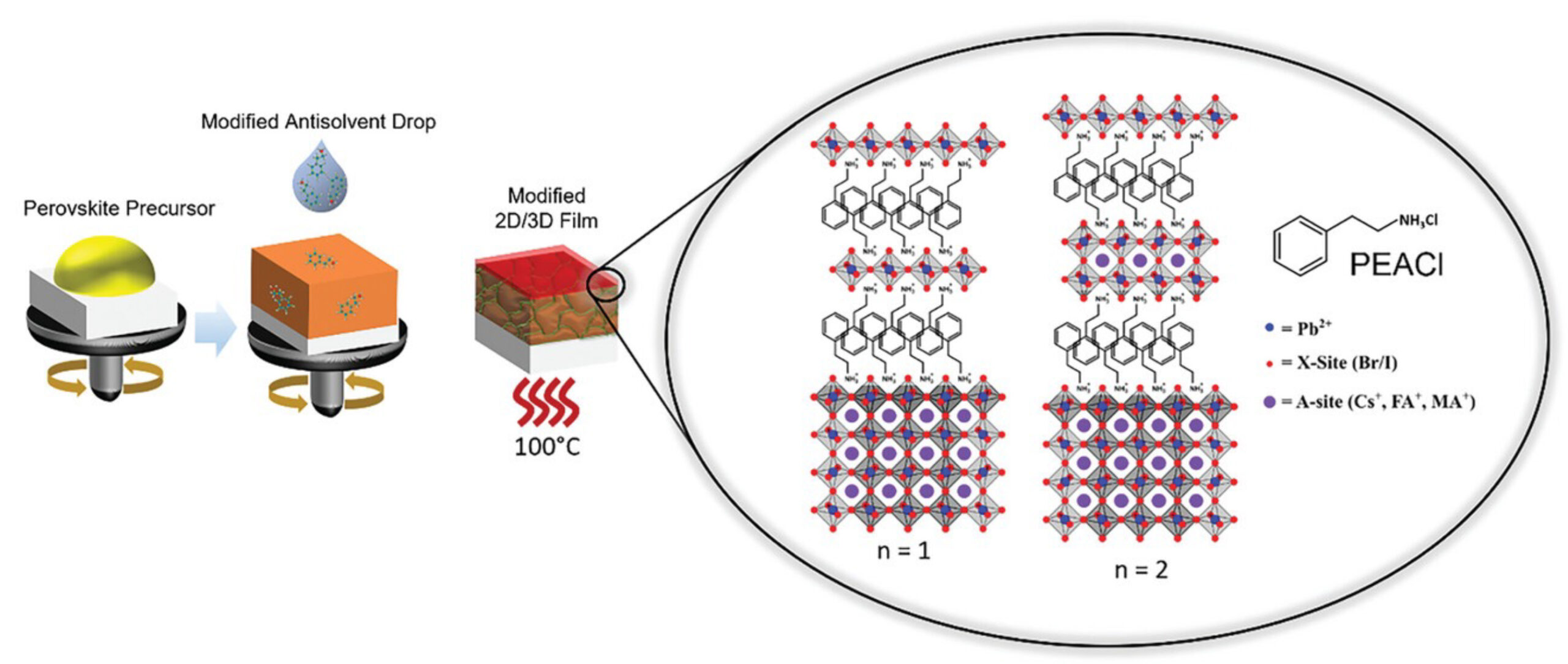
Promising perovskites
Perovskites—crystalline materials with the potential to revolutionize solar technology—have numerous advantages over traditional silicon-based solar panels. They absorb light much more broadly and efficiently, can be made 100 times thinner, perform well with material impurities, and require lower temperatures to process. Despite their advantages, however, perovskite solar panels lack the stability and durability to last for 20 to 30 years in all kinds of weather.
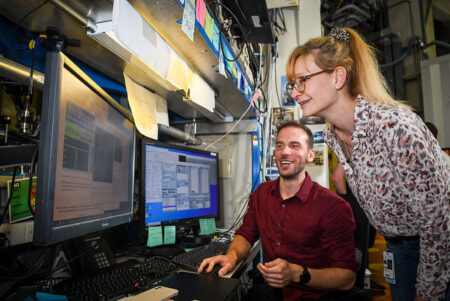
Recently, scientists from the University of Stuttgart developed a new process for making perovskite photovoltaic films. The resulting cells are very efficient and stable, but it was not clear why the process worked so well. To find out, the researchers collaborated with a team from Berkeley Lab’s Molecular Foundry, who had developed multimodal tools for in situ monitoring of photovoltaic materials at an ALS beamline. There, the researchers could watch the formation of perovskite films in real time, from initial building blocks to final state. The insights from this work can help address key barriers to commercializing this promising technology.
Protective layer of bulky molecules
One approach to improving durability involves fabricating a perovskite solar cell and then depositing a large, “bulky” organic molecule—in this case, phenethylammonium chloride (PEACl)—on its surface. Researchers studying this process have found that PEACl forms hydrophobic (quasi-)2D structures at the surface of the perovskite film that are effective at keeping out moisture.
The Stuttgart team improved the process by combining perovskite crystallization and PEACl deposition into a single step. The simplified process enables researchers to make cells more durable and efficient and of more consistent quality than cells made the traditional way. Longer term, the process can potentially reduce the costs and energy consumption of perovskite cell manufacturing. To discover why the process works, the researchers turned to the Molecular Foundry and the ALS.
Slower growth, higher quality
The researchers studied two types of samples. In the first, PEACl was deposited on an already-crystallized perovskite surface. In the second, the PEACl was combined with an antisolvent (a solidifying agent) before crystallization. At ALS Beamline 12.3.2, a custom-made analytical chamber allowed for the spin-coating fabrication process and two simultaneous measurements: grazing-incidence wide-angle x-ray scattering (GIWAXS), to follow structural changes, and laser-based photoluminescence (PL), to track optical response. A third technique, white-light absorbance, was conducted at the Molecular Foundry.
The researchers correlated the data streams from the three techniques to produce a detailed understanding of the evolution of the crystal structure of the samples. The data revealed that the crystals in the PEACl-treated samples grew more slowly and were of higher quality, with the protective PEACl-containing hydrophobic (quasi-)2D structures still exclusively forming at their surface. The team believes these findings explain the cells’ improved efficiency and stable operation as measured for over a month.
The researchers envision several possible avenues for future exploration, including treating perovskites with other molecules to see how they affect durability and efficiency. They would also like to monitor the cell’s stability over a longer period of operation and under more realistic environmental conditions. The idea would be to understand which material combinations improve the cell’s stability and thus can extend its lifetime.
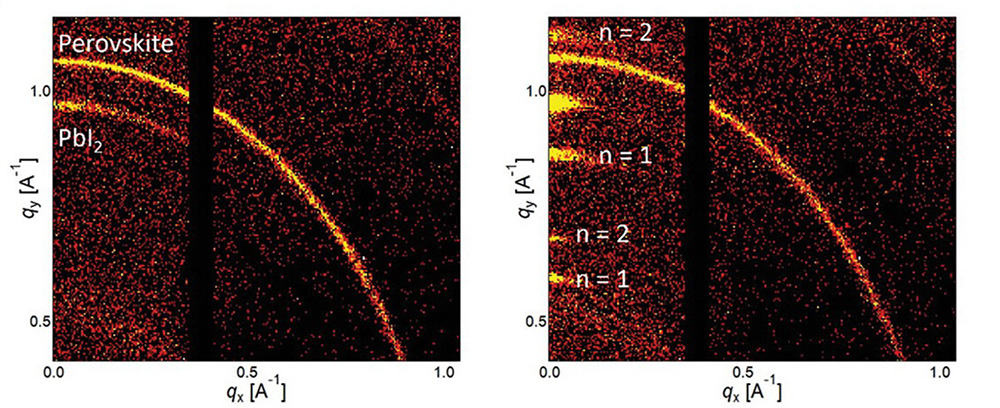
Contacts: Carolin Sutter-Fella and Tim Kodalle
Researchers: T. Kodalle, M. Goudreau, and C.M. Sutter-Fella (Berkeley Lab); M.M. Byranvand, C. Das, and M. Saliba (University of Stuttgart, Germany, and Forschungszentrum Jülich, Germany); R. Roy, M. Zohdi, and M. Rai (University of Stuttgart, Germany); M. Kot (Brandenburg University of Technology Cottbus-Senftenberg, Germany); S. Briesenick (Berkeley Lab and McGill University, Canada); N. Tamura (ALS); J.I. Flege (Brandenburg University of Technology Cottbus-Senftenberg, Germany); and W. Hempel (Centre for Solar Energy and Hydrogen Research Baden-Württemberg, Germany).
Funding: German Research Foundation (DFG); US Department of Energy, Office of Science, Basic Energy Sciences program (DOE BES); UC Berkeley and Berkeley Lab; Centre for the Development of Industrial Technology (CDTI), Spain; State Research Agency (AEI), Spain; Federal Ministry for Economic Affairs and Climate Action (BMWK), Germany; Israel Ministry of Energy; European Research Council; and Federal Ministry of Education and Research (BMBF), Germany. Operation of the ALS and Molecular Foundry is supported by DOE BES.
Publication: T. Kodalle, M.M. Byranvand, M. Goudreau, C. Das, R. Roy, M. Kot, S. Briesenick, M. Zohdi, M. Rai, N. Tamura, J.I. Flege, W. Hempel, C.M. Sutter-Fella, and M. Saliba, “An Integrated Deposition and Passivation Strategy for Controlled Crystallization of 2D/3D Halide Perovskite Films,” Adv. Mater. 36 2309154 (2024), doi:10.1002/adma.202309154.
Adapted from the Berkeley Lab press release, “New Insights Lead to Better Next-Gen Solar Cells.”
ALS SCIENCE HIGHLIGHT #504